Home > Medical Research Archives > Issue 149 > Evaluation of Pulmonary Functions by Spirometry after Stereotactic Body Radiotherapy in Lung Tumors
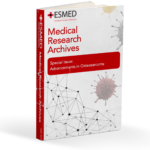
Published in the Medical Research Archives
Jun 2023 Issue
Evaluation of Pulmonary Functions by Spirometry after Stereotactic Body Radiotherapy in Lung Tumors
Published on Jun 26, 2023
DOI
Abstract
Introduction: To evaluate changes in pulmonary function by spirometry after stereotactic body radiotherapy (SBRT).
Methods: A single-center, retrospective study was performed which analyzed lung function after SBRT from January 1, 2015 to January 31, 2020. Eligible patients were ≥18 years of age, with early-stage lung cancer or lung metastases ≤5 cm and Karnofsky performance status (KPS) >70. Patients were excluded if they had a history of non-infectious pneumonitis or interstitial lung disease. Clinical cases were discussed in a multidisciplinary tumor board, and the patients were classified as surgically resectable but medically inoperable. Mixed-effects models were used to evaluate changes in forced expiratory volume in 1 (FEV1), forced vital capacity (FVC) and diffusing capacity of the lungs for carbon monoxide (DLCO) at 3, 6, 12, and 24 months after SBRT.
Results: Fifty-two patients underwent SBRT. Median follow-up spirometry post-SBRT was 12 months (range, 12-24). No significant differences were observed during the first year in both liters and the percentage of predicted FEV1 evolution. A gain of 5.9% [95% CI, 2.3; 9.6 percent (p=0.0014)] was beheld after 24 months post-SBRT. Although, no significant difference has been viewed when we analyze it in liters with an FEV1 value 24 months post-SBRT of 1.57 liters [95% CI, 1.56; 1.59 liters (p=0.848)]. There are no differences when we analyze FVC evolution according to liters during the first two years post treatment. Finally, no differences were observed when analyzing DLCO evolution according to percentage or as ml/min/kPa.
Conclusions: SBRT in primary lung tumors or pulmonary metastases does not negatively influence pulmonary function assessed by spirometry at least in the first two years after treatment.
Author info
INTRODUCTION
To date, lung cancer continues to be one of the most commonly diagnosed tumors and the leading cause of cancer deaths1. The high prevalence of smoking in the general population and inadequate tobacco control policies contribute as a major factor in the development of lung cancer and chronic obstructive pulmonary disease (COPD).
Early-stage in non-small cell lung cancer (NSCLC) represents approximately 30 percent of all cases2. The standard of care for patients with stage I-IIA NSCLC according to American Joint Committee on Cancer (AJCC) 8th edition is surgical resection with systematic or lobe-specific lymph node dissection3. Stereotactic body radiation therapy (SBRT) has been shown to be a treatment as effective as surgery. Several publications suggest that SBRT outcomes are similar to those of surgery, regarding disease control4-9. Similarly, for decades metastasectomy has been recognized as the ideal treatment for lung metastases in oligometastatic patients. Based on the results of early-stage NSCLC, SBRT in the setting of the metastatic patient is gaining ground over surgery, especially in selected patients based on good performance status and slow pace of tumor progression10,11.
In either scenario, the impact of treatment on lung function is of particular interest. The consequences of any of these therapeutic approaches could result in a detriment of lung capacity that translates into a negative impact on quality of life, especially in elderly patients with primary tumors and tobacco-associated lung pathology. In metastatic patients, many of them without previous pulmonary pathology, the interest lies in that with the increase in survival gained in most primary tumors, the frequent need for multiple treatments at pulmonary level has also increased.
Stereotactic body radiotherapy and surgical procedures are treatments with radical intent, but complications are somewhat different between them. In most surgical series, forced expiratory volume in 1 (FEV1) and diffusing capacity of the lungs for carbon monoxide (DLCO) on respiratory function tests were shown to be predictors of postoperative complications12-15. At our center, we questioned whether these parameters could be equally predictive of complications or responses in patients treated with SBRT for primary tumor or lung metastases.
Many working groups have published their results in patients treated with SBRT for primary lung tumors or for lung metastases. These results have allowed us to demonstrate the good tolerance of the technique and the low rates of serious complications. The publications aimed at showing changes in spirometric parameters are however more varied and in most of the publications masked by other treatments such as various courses of SBRT or systemic or immunotherapy treatments that also have an impact on lung function.
Therefore, the aim of this retrospective study was to perform a comprehensive follow-up of pulmonary function after curatively intended SBRT, in patients without disease progression and for two years to try to reduce the impact of increasing age in this respect. The present report provides our outcomes in terms survival and lung functions until the progression.
METHODS
The study population was collected from an evaluation of our institutional database to identify patients with T1-T2 /N0 NSCLC (American Joint Committee on Cancer, 8th Edition, 2020) or lung metastases smaller than 5 cm, treated with SBRT with radical intention. Pulmonary function tests (PFT) before and after SBRT were collected by spirometry. Eligible patients were 18 years of age or older with a Karnofsky Performance Status (KPS)
>70. Ultra-central, central and peripheral tumors were included. In addition, all patients included had PFT pre-treatment and at least one spirometry during the first two years of follow-up, and PFTs should have been performed while their pulmonary pathology was stable. New primary tumors in patients who were previously operable were included. Histological diagnosis was necessary for patients without high risk of tumor samples. At diagnosis and for each patient, an evaluation in the tumor board committee was carried out to decide the most indicated treatment. Patients were suitable for radiotherapy when for medical reasons the patient was considered to be inoperable or if the patient refused to undergo surgery. Synchronous multiple lung tumors, non-tolerance to the SBRT immobilization, patients who had received concurrent chemotherapy or immunotherapy, prior thoracic radiotherapy, and patients without spirometry during follow-up were excluded.
Treatment
Patients were placed in a Stereotactic Body Frame (Qfix AltaTM Multipurpose Device), using a vacuum pillow for reproducible immobilization. Lateral and midline positioning marks were permanently applied. Given the absence of fluoroscopy in the computed tomography (CT)- simulation, we used abdominal pressure in targets located in the lower lobes or in upper lobe tumors with motion greater than 1 cm, as long as the patient tolerated the compression. 4D PET-CT images were used for treatment planning until March 2019 and thereafter treatment planning would be carried out with 4D-CT. The gross tumor volume (GTV) delineation was worked in the lung window. An internal target volume (ITV) was contoured on each phase of the 4D-PET or 4D-CT depending on the planning date, and a union of the GTVs was used to create a composite ITV. Finally, the planning target volume was obtained by a 0.5 cm uniform expansion from the internal target volume. Patients were treated in a Truebeam linear accelerator. For treatment verification, following our image-guided radiotherapy protocol (IGRT), a 3D or 4D CBCT was used to compare with the planning CT.
Following our institutional Lung SBRT protocol at that time, patients with centrally located lesions (a tumor within 2 cm to any mediastinal critical structure) received a total radiation dose of 50-55 Grey (Gy) in five fractions or 60 Gy in eight fractions. For peripherally located tumors (lesions 2 cm away to any mediastinal critical structures) 54- 60 Gy in three fractions was prescribed or 50-55 Gy in five fractions. Other ablative dose regimens with a biologically effective dose (BED) >100 Gy (calculated with tumor α/β=10) were also allowed. Regardless of location, the most important criterion for considering the appropriate treatment schedule was whether the dose-volume constraints of normal tissue could be met.
Response and toxicity evaluation
Follow-up clinical examinations were scheduled at 1, 3, and 6 months after SBRT, and every 6 months thereafter in all patients. CT or whole-body PET scan was performed to assess for local relapse and metastatic spread, and a spirometry was set to evaluate lung function at 3, 6, 12, and 24 months after radiation therapy. Local relapse was defined as a tumor recurrence within the planning target volume (PTV), regional failure as outside PTV in the same lung lobe or mediastinal nodal, and metastases distant as appearance in other initial lung lobe or distant organ sites. The end of follow-up was determined as disease progression leading to the need for further treatment with impact on lung function, death from any cause or the closure of the study on 31 January 2020. Treatment toxicity was recorded according to the Common Terminology Criteria for Adverse Events (CTCAE) version 5.0.
Outcomes and statistical analysis
Results are expressed as frequencies and percentages for qualitative variables and as median (interquartile range, IQR) for quantitative variables. Fixed effects in a mixed-model regression analysis were used to evaluate changes in FEV1 at 3, 6, 12, and 24 months after SBRT. The Kaplan-Meier estimator was used for survival analysis of the whole group using KPS as an independent variable. A significant difference in all two-sided p-values was considered <0.05.
Stata version 15.1 was used to analyze the database. This study was reviewed and approved by the Comitè Ètic d’Investigació́ Clínica (CEIC) of Vall d’Hebron University Hospital. Informed consent was not required according to national and institutional legislation.
RESULTS
From 1 January 2015 to 31 January 2020, ninety-two patients underwent pulmonary SBRT at our center; fifty-two (N=52) patients were included in our analysis of which forty-four were treated for primary lung tumor and the other eight patients for pulmonary metastases. The clinical and demographic characteristics included in the present study are shown in Table 1. Median follow-up spirometry after treatment was 12 months (IQR, 12- 24). The evolution of median values for spirometry before SBRT and 3, 6, 12, and 24 months post-SBRT were summarized in Table 2.
Table 1. Demographic and clinical characteristics:
Table 2. Pulmonary function
a Pulmonary function was expressed in absolute values (liters or mmol/min/kPa) and percentage (%) prior to SBRT and 3, 6, 12, and 24 months after SBRT.
b Data are presented as the median (interquartile range).
Likewise, a mixed-model regression analysis was performed to predict changes in values of pulmonary functions tests as FEV1 which is represented in Figure 1A-B. The intercept which FEV1 value before treatment was 1.58 liters (95% CI 1.41; 1.74 liters) and 59.06 percent (95% CI 52.9; 65.1 percent). No significant differences were observed during the first year in both liters and percentage of predicted. A gain of 5.9 percent [95% CI 2.3; 9.6 percent (p=0.0014)] was beheld after 24 months post-SBRT (figure 1A). Although, no significant difference has been viewed when we analyze it in liters with a FEV1 value 24 months post- SBRT of 1.57 liters [95% CI 1.56; 1.59 liters (p=0.848)] (figure 1B).
Forced vital capacity (FVC) evolution is also analyzed using mixed-effects models for linear regression which is shown in figure 1B-C with an intercept of 63.6 percent (95% CI 58.4; 68.9 percent) and 2.41 liters (95% CI 2.20; 2.62 liters). FVC increases by 4.4 (95% CI 1.1; 8.6; p=0.042), 4.9 (95% CI 1.1; 9.2; p=0.028), and 11.3 percent (95% CI 6.9; 15.7 (p=0.0000) at 3, 12, and 24 months, respectively. There are no differences when we analyze FVC evolution according to liters during the first two years post treatment. At 3, 6, 12 and 24 months we observed a stabilization in the FVC evolution with respect to the intercept of 0.10 liters [95% CI -0.3; 0.23 liters (p=0.1459)], -0.03 liters [95% CI -0.17; 0.11 liters (p=0.6973)], 0.03 liters [95% CI -0.08; 0.15 liters (p=0.5817)] and 0.05 liters [95% CI -0.13; 0.14 liters (p=0.0.9452)], respectively.
Finally, no differences were observed when analyzing DLCO evolution according to percentage or as results in ml/min/kPa (figure 1E-F). An intercept of 54.9 percent (95% CI 49.0; 61.0 percent) and 4.46 mmol/min/kPa (95% CI 3.96; 4.95) was obtained in the mixed-effects model. The resulting data from the DLCO evolution in the analysis of percentage of predicted at 3, 6, 12, and 24 months are 2.3 [95% CI -2.9; 7.5 percent (p=0.3976)], 0.2 [95% CI -5.5; 5.7 percent (p=0.9577)], 0.4 [95% CI -4.8; 5.6 percent (p=0.8673)], and -4.7 percent [95% CI -10.0; 0.5 percent (p=0.0765)], respectively (figure 1C). Regarding the evolution of DLCO in mmol/min/kPa, the following outcomes were observed of 0.21 [95% CI -0.28; 0.71 mmol/min/kPa (0.4006)], - 0.39 [95% CI -0.93; 0.15 mmol/min/kPa (p=0.1566)], -0.05 [(95% CI -0.52; 0.43 mmol/min/kPa (p=0.8541)], and -0.047 mmol/min/kPa [95% CI -0.97; 0.04 mmol/min/kPa (p=0.0699)], respectively.
Figure 1. Mixed-Model regression analysis of FEV1, FVC and DLCO evolution after lung SBRT.
The Kaplan-Meier method was used to estimate overall survival (OS) and progression-free survival (PFS) in the whole group. Median OS was 4.55 years (range, 3.23 - not reached). OS analysis showed 96.15% (95% CI 85.48; 99.02), 86.10% (95% CI 73.01; 93.13), and 45.06% (95% CI 25.20; 63.07) at 1, 2 and 5 years, respectively (figure 2A). Median PFS was not reached. One-, 2- and 5-year PFS rate was 98.00% (95% CI 86.64; 99.72), 91.35% (95% CI 78.51; 96.67), and 59.54% (95% CI 41.33; 73.77) (figure 2B).
Figure 2. Overall survival (OS) and progression free-survival (PFS) after SBRT.
No significant differences were observed in OS (p=0.3524) and PFS (p=0.1733) when analyzed by early-stage and advanced stage. Early-stage cases showed at 1, 2 and 5-year OS rate of 97.73% (95% CI 84.94; 99.68), 85.75% (95% CI 70.95; 93.35), and 47.68% (95% CI 25.74; 66.75), and at 2 and 5-year PFS rate of 97.50% (95% CI 83.55; 99.64), and 81.22% (95% CI 59.62; 91.97), respectively. While advanced stages showed at 1, 2 and 5-year OS rate of 87.50% (95% CI 38.70; 98.14), 87.50% (95% CI 38.70; 98.14), and 38.89% (95% CI 6.30; 72.42), and 2- and 5-year PFS rate of 100% (95% CI NR; NR), and 66.67% (95% CI 19.46; 90.44), respectively.
Twenty (38.46%) patients died at the end of follow-up. However, only six (11.54%) deaths were due to cancer. Table 3 summarizes the clinical characteristics and causes of non-cancer deaths.
A total of 7 (13.46%) patients presented acute toxicity of which four (7.69%) of them developed radiation-induced dyspnea grade 2, two (3.85%) patients experienced cough grade 2 and one (1.92%) patient presented a chest pain grade 1. No acute toxicity grade 3 or higher was reported. After survival analysis, patients who developed radiation-induced dyspnea had worst survival rate compared to those who did not develop it (p=0.0055). At 2-year OS rate in radiation-induced dyspnea cases was 50.00% (95% CI 5.78; 84.49), and at 2-year OS rate in cases who did not develop radiation-induced dyspnea was 88.76% (95% CI 75.04; 95.17).
Univariate Cox proportional hazards regression analysis was carried out according to stage and radiation-induced dyspnea grade 2 or more. Advanced-stage (IV) was not significantly associated with OS (hazard ratio [HR], 1.69; 95% CI, 0.55; 5.15; p=0.3578, with early-stage as reference group) or PFS (HR 2.18; 95% CI, 0.69; 6.90; p=0.1841, with early-stage as reference group). Patients who did not present radiation- induced dyspnea grade 2 or more were significantly associated with a 88% decreased risk of mortality (HR 0.12; 95% CI, 0.02; 0.65; p=0.0198). Clinical and dosimetric characteristics of the patients who developed pneumonitis grade 2 are shown in table 4.
DISCUSSION
Care of the respiratory function of patients with primary or oligometastatic lung tumors is critical as most of them have cardiorespiratory comorbidities. Even those patients without these comorbidities have a high likelihood of needing repeated lung parenchymal treatments, so it is essential to maintain adequate respiratory function. In this sense, the assessment of functional tests and their evolution after specific oncological treatments is a challenge. The main objective of our study was to report the evolution of lung function by spirometry and lung toxicity after two years post- SBRT for early-stage NSCLC or lung metastases in oligometastatic patients of any primary.
While the incidence of NSCLC over the years has become more balanced between men and women16, in our cohort 80% were men, although this is also due to the age of our sample and the inclusion of metastatic patients of any primary in the analysis. Surprisingly, only about half of the patients in our study were classified as COPD, although presumably, this percentage may be higher; this assumption is due to the fact that only 15% of patients denied any previous or current relationship with smoking, and that in our setting only patients with persistent respiratory symptoms are evaluated by Pneumology and only up to that point are they classified as COPD in their medical history. Nonetheless, respiratory pathology was the main reason for denying surgery to more than half of the patients (59.6%), unlike some series where the main comorbidity that precludes surgery is cardiovascular17,18.
The end of follow-up was defined as disease progression, death or the end of the study. In this way, we diminished the role of other factors that can also alter lung function, beyond age. Follow-up pulmonary function tests were performed during the first two years of treatment with at least one spirometry during follow-up, however, it is noteworthy that 53.8% 34.6%, 50% and 48.1% of the sample had functional tests 3, 6, 12 and 24 months after SBRT, respectively. The difficulty of the technique in elderly patients and the onset of the COVID pandemic, conditioned to some extent the possibility of having more PFRs per patient during follow-up.
Forced expiratory volume in 1 (FEV1) values below 70% and 1 liter were 69% and 15.4%, respectively. DLCO values were not performed in 21% of the study sample prior to radiotherapy. The reason for this lack of data in DLCO is caused by the fact that with FEV1 values below 50% or 1 liter, measurement of DLOC is not feasible. In our results, during follow-up, non-significant changes in FEV1, FVC and DLCO were observed. These results are consistent with the majority of the literature on the matter. An Indiana University study of dose escalation in SBRT19 demonstrated that, after treatment, a total of 10 of 37 patients had a decrease of at least 10% of predicted in at least one measured value of lung function (i.e. FEV1, FVC, DLCO or Po2) but with a return to baseline at long- term follow-up. The same authors in their phase II protocol20 documented only a small decrease in DLCO of 1.11 mg/min/mm Hg/a but revealed no change in FEV1. Ohashi et al21 detailed stability of FEV1 and a discrete increase in DLCO in a small cohort of Asian patients. The RTOG 0236 study22 showed no change in FVC, FEV1 or FEV1% at 2 years after SBRT in 55 patients analyzed. Takeda et al.23 published a cohort of 141 patients with a median follow-up of 21.0 months after SBRT and found significant decreases in FVC and FEV1, but these were not significant in patients with COPD. Shinya et al.24 evaluated 70 patients and revealed that FVC decreased from 2.67 to 2.51 L (p < 0.01) and mean FEV1 decreased from 1.80 to 1.72 L (p < 0.01).
The impact of the location of the lesion to be treated and patients with very low results of PFT are important issues that are often raised but remain unresolved, beyond question. Stephans et al.25 reported a patient with a FEV1 relative value as low as 15% who achieved an adequate tumor control without complications after SBRT. In contrast to other publications22,25, in our study 32% of treatments were centrally localized and treated with a curative intent. We may hypothesize that localization, as long as the restriction dose to organs at risk is guaranteed, should not generate an increased risk of toxicity in PFTs. Larger multicenter databases may provide a larger set of patients on which to base a PFT threshold especially for central tumors.
When analyzing overall survival, the median OS was 4.5 years and the median PFS was not reached. A survival analysis also is shown in patients who developed radiation-induced dyspnea who had a worst survival rate compared to those who did not. This outcome may support the result from RTOG 023622 that poor baseline PFT did not predict drop off in overall survival after SBRT. Other studies also reported similar results regarding the safety of SBRT in patients with poor lung function20,26. In our sample, 6 of the non-tumor- related deaths were due to pneumonia, 3 of them bacterial and the rest related to COVID or influenza. With the profile of our patients, these results are not surprising, but it is noteworthy that none of the deaths occurred within the first 12 months post-treatment and therefore it seems less reasonable to attribute to post-treatment toxicity, indeed because no toxicities of grade 3 or higher were reported.
Radiation pneumonitis is the most feared adverse effect after SBRT for lung cancer. The rate of radiation pneumonitis grade >2 varies among different studies between 1.8-14.5%7,27-30. The present study focused on patients with lung cancer or metastases with or without previous lung function impairment, and only 7% of patients developed ≥2 radiation pneumonitis. Given the low numbers involved it is difficult to draw reliable conclusions in this respect. In table 4 we can see some clinical and dosimetric details of these patients, highlighting that only 1 patient had a central location and that half of the patients were not classified as COPD. These findings suggest that the incidence of severe radiation pneumonitis after SBRT in lung cancer patients with poor lung function is similar to that of patients with normal lung function. With similar findings, Guckenberger et al.31 analyzed patients with NSCLC treated with SBRT to assess the influence of pre-treatment lung function on lung toxicity; no significant associations were observed between any of the pre-treatment lung function parameters and the risk of radiation pneumonitis grade ≥2.
This study had some limitations. The most important of all is the poor follow-up on respiratory function tests. In early-stage patients, spirometries are not routinely performed as part of oncological follow-up, let alone in patients treated for pulmonary metastases. This leads to an important deficit in the assessment of chronic toxicity. In our center, PFT is requested as part of the pneumology follow-up in patients with severe COPD or in patients with suspected severe radiation toxicity. Other relevant limitations are the retrospective design and the fact that our study reflects the clinical practice of a single center, so its interpretation should be understood with caution.
CONCLUSIONS
These results support the safety of SBRT in early-stage NSCLC and pulmonary oligometastases, even for those patients with a history of impaired lung function. In our center no patients were denied treatment based on pulmonary function and there was no significant decline values in PFTs noted with treatment. These findings furtherance the idea that there is no limit in respiratory function tests to deny treatment of small lung lesions with SBRT.
CONFLICTS OF INTEREST STATEMENT
No conflict of interest.
FUNDING STATEMENT
No disclosure of funding.
REFERENCES
1. American Cancer Society. Cancer Facts & Figures 2021. Accessed October 15, 2022. https://cancer.org/research/cancer-facts- statistics/all-cancer-facts-figures/cancer-facts- figures-2021.html.
2. Groome PA, Bolejack V, Crowley JJ, et al. The IASLC Lung Cancer Staging Project: validation of the proposals for revision of the T, N, and M descriptors and consequent stage groupings in the forthcoming (seventh) edition of the TNM classification of malignant tumours. J Thorac Oncol. 2007;2(8):694-705.
3. Rami-Porta R, Wittekind C, Goldstraw P. Complete resection in lung cancer surgery: proposed definition. Lung Cancer. 2005;49(1):25-33.
4. Onishi H, Shioyama Y, Matsumoto Y, et al. Stereotactic body radiotherapy in patients with lung tumors composed of mainly ground-glass opacity. J Radiat Res. 2020;61(3):426-30.
5. Matsuo Y, Chen F, Hamaji M, et al. Comparison of long-term survival outcomes between stereotactic body radiotherapy and sublobar resection for stage I non-small-cell lung cancer in patients at high risk for lobectomy: A propensity score matching analysis. Eur J Cancer. 2014;50(17):2932-8.
6. Chang JY, Senan S, Paul MA, et al. Stereotactic ablative radiotherapy versus lobectomy for operable stage I non-small-cell lung cancer: a pooled analysis of two randomised trials. Lancet Oncol. 2015;16(6):630-7.
7. Nagata Y, Hiraoka M, Shibata T, et al. Prospective Trial of Stereotactic Body Radiation Therapy for Both Operable and Inoperable T1N0M0 Non-Small Cell Lung Cancer: Japan Clinical Oncology Group Study JCOG0403. Int J Radiat Oncol Biol Phys. 2015;93(5):989-96.
8. Shibamoto Y, Hashizume C, Baba F, et al. Stereotactic body radiotherapy using a radiobiology-based regimen for stage I non- small-cell lung cancer: five-year mature results. J Thorac Oncol. 2015;10(6):960-4.
9. Miyakawa A, Shibamoto Y, Baba F, et al. Stereotactic body radiotherapy for stage I non- small-cell lung cancer using higher doses for larger tumors: results of the second study. Radiat Oncol. 2017;12(1):152.
10. Hellman S, Weichselbaum RR. Oligometastases. J Clin Oncol. 1995;13(1):8-10.
11. Palma DA, Olson R, Harrow S, et al. Stereotactic ablative radiotherapy versus standard of care palliative treatment in patients with oligometastatic cancers (SABR- COMET): a randomised, phase 2, open-label trial. Lancet. 2019;393(10185):2051-8.
12. Cao C, Louie BE, Melfi F, et al. Impact of pulmonary function on pulmonary complications after robotic-assisted thoracoscopic lobectomy. Eur J Cardiothorac Surg. 2020;57(2):338-42.
13. Burt BM, Kosinski AS, Shrager JB, Onaitis MW, Weigel T. Thoracoscopic lobectomy is associated with acceptable morbidity and mortality in patients with predicted postoperative forced expiratory volume in 1 second or diffusing capacity for carbon monoxide less than 40% of normal. J Thorac Cardiovasc Surg. 2014;148(1):19-28, dicussion 28-29.e1.
14. Kearney DJ, Lee TH, Reilly JJ, DeCamp MM, Sugarbaker DJ. Assessment of operative risk in patients undergoing lung resection. Importance of predicted pulmonary function. Chest. 1994;105(3):753-9.
15. Ferguson MK, Little L, Rizzo L, et al. Diffusing capacity predicts morbidity and mortality after pulmonary resection. J Thorac Cardiovasc Surg. 1988;96(6):894-900.
16. Siegel RL, Miller KD, Fuchs HE, Jemal A. Cancer statistics, 2022. CA Cancer J Clin. 2022;72(1):7-33.
17. Ambrogi V, Pompeo E, Elia S, Pistolese GR, Mineo TC. The impact of cardiovascular comorbidity on the outcome of surgery for stage I and II non-small-cell lung cancer. Eur J Cardiothorac Surg. 2003;23(5):811-7.
18. Kravchenko J, Berry M, Arbeev K, Lyerly HK, Yashin A, Akushevich I. Cardiovascular comorbidities and survival of lung cancer patients: Medicare data based analysis. Lung Cancer. 2015;88(1):85-93.
19. Timmerman R, Papiez L, McGarry R, et al. Extracranial stereotactic radioablation: results of a phase I study in medically inoperable stage I non-small cell lung cancer. Chest. 2003;124(5):1946-55.
20. Henderson M, McGarry R, Yiannoutsos C, et al. Baseline pulmonary function as a predictor for survival and decline in pulmonary function over time in patients undergoing stereotactic body radiotherapy for the treatment of stage I non- small-cell lung cancer. Int J Radiat Oncol Biol Phys. 2008;72(2):404-9.
21. Ohashi T, Takeda A, Shigematsu N, et al. Differences in pulmonary function before vs. 1 year after hypofractionated stereotactic radiotherapy for small peripheral lung tumors. Int J Radiat Oncol Biol Phys. 2005;62(4):1003- 8.
22. Stanic S, Paulus R, Timmerman RD, et al. No clinically significant changes in pulmonary function following stereotactic body radiation therapy for early- stage peripheral non-small cell lung cancer: an analysis of RTOG 0236. Int J Radiat Oncol Biol Phys. 2014;88(5):1092-9.
23. Takeda A, Kunieda E, Ohashi T, et al. Severe COPD is correlated with mild radiation pneumonitis following stereotactic body radiotherapy. Chest. 2012;141(4):858-66.
24. Takemoto S, Shibamoto Y, Hashizume C, et al. Changes in pulmonary function and their correlation with dose-volume parameters in patients undergoing stereotactic body radiotherapy for lung cancer. J Radiat Res. 2021;62(2):338-45.
25. Stephans KL, Djemil T, Reddy CA, et al. Comprehensive analysis of pulmonary function Test (PFT) changes after stereotactic body radiotherapy (SBRT) for stage I lung cancer in medically inoperable patients. J Thorac Oncol. 2009;4(7):838-44.
26. Stone B, Mangona VS, Johnson MD, Ye H, Grills IS. Changes in Pulmonary Function Following Image-Guided Stereotactic Lung Radiotherapy: Neither Lower Baseline Nor Post-SBRT Pulmonary Function Are Associated with Worse Overall Survival. J Thorac Oncol. 2015;10(12):1762-9.
27. Timmerman R, Paulus R, Galvin J, et al. Stereotactic body radiation therapy for inoperable early stage lung cancer. JAMA. 2010;303(11):1070-6.
28. Liu Y, Wang W, Shiue K, et al. Risk factors for symptomatic radiation pneumonitis after stereotactic body radiation therapy (SBRT) in patients with non-small cell lung cancer. Radiother Oncol. 2021;156:231-8.
29. Saha A, Beasley M, Hatton N, et al. Clinical and dosimetric predictors of radiation pneumonitis in early-stage lung cancer treated with Stereotactic Ablative radiotherapy (SABR) - An analysis of UK\\\'s largest cohort of lung SABR patients. Radiother Oncol. 2021;156:153-9.
30. Kong FS, Moiseenko V, Zhao J, et al. Organs at Risk Considerations for Thoracic Stereotactic Body Radiation Therapy: What Is Safe for Lung Parenchyma. Int J Radiat Oncol Biol Phys. 2021;110(1):172-87.
31. Guckenberger M, Kestin LL, Hope AJ, et al. Is there a lower limit of pretreatment pulmonary function for safe and effective stereotactic body radiotherapy for early-stage non-small cell lung cancer. J Thorac Oncol. 2012;7(3):542-51.
Author Area
Have an article to submit?
Submission Guidelines
Submit a manuscript
Become a member