Home > Medical Research Archives > Issue 149 > Cerebrospinal CNS-Leaks: State of the Art in Imaging Diagnosis with Special Focus on Intrathecal MRI Paramagnetic Contrast Agents Procedures
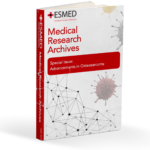
Published in the Medical Research Archives
Jun 2023 Issue
Cerebrospinal CNS-Leaks: State of the Art in Imaging Diagnosis with Special Focus on Intrathecal MRI Paramagnetic Contrast Agents Procedures
Published on Jun 26, 2023
DOI
Abstract
MR imaging has proved invaluable in anatomic depiction of the cerebrospinal fluid (CSF) spaces and the surrounding neural and non-neural tissue, although there are still some clinical situations (i.e., cases of CSF-flow alterations, communicating or non-communicating cyst masses bordering CSF pathways, or craniospinal CSF leaks) in which further imaging tests may be required for a definitive diagnosis.
This paper will review the state-of-the-art imaging in these processes, including Radionuclide Cisternography, plain Computed Tomography (CT) and enhanced-CT Cisternography/ myelography, as well as Magnetic Resonance Imaging (MRI) and contrast-enhanced Cisternography/myelography, emphasizing the latest CT and MR imaging refinement advances and proposing tailored specific approaches for two well-established clinical syndromes, namely CSF rhinorrhea and intracranial hypotension syndrome.
Author info
INTRODUCTION
Computed Tomography (CT) and Magnetic Resonance Imaging (MR) are currently the preferred techniques for the diagnosis of morphological diseases involving the CNS. Intravenous contrast materials may add important information, particularly regarding abnormalities in blood-brain-barrier integrity which is frequently disturbed by neoplastic and inflammatory illness. MRI is superior to CT in depicting many lesions. Advantages of MRI over CT relays on its superior contrast resolution, superb anatomic display, and absence of radiation exposure. For these reasons MRI is the standard of imaging reference in processes involving the brain and spinal cord.
MRI has also proved invaluable in the anatomic depiction of the cerebrospinal fluid (CSF) containing spaces surrounding neural and non- neural tissues. However, there are still some clinical situations (i.e., cases of CSF flow alterations, communicating or non-communicating cyst masses bordering CSF pathways, or craniospinal CSF leaks) which may require further imaging tests for definitive diagnosis and there are still a number of conditions where CSF contrast enhancement is beneficial, including assessment of the subarachnoid spaces, abnormal CSF collections and leaks, or as a mean to study CSF dynamics. Both radioisotope cisternography (RC) and CT with intrathecal water- soluble iodinated contrast media have been used for this purpose but have limitations and use ionizing radiation.
Cisternography is defined as imaging of the cerebrospinal system and associated structures after intrathecal administration of contrast material into the subarachnoid space5,6. Cisternographic studies have been in use for at least 50 years for the evaluation of abnormalities of the intracranial CSF-filled spaces, cranium base, and vertebral column. Cisternography is called “myelography” if it is dedicated to the spinal canal5,6. In this review,paramagnetic.
The main limitations of RC are lack of cross-sectional images and lower spatial resolution. CT has overcome these limitations; however, both contrast- enhanced CT cisternography (CE-CTC) and RC involve radiation exposure, which is a more important factor to consider because cisternographic studies often require consecutive or multiple acquisitions5-10.
Non-contrast High-Resolution T2-weighted MR Cisternography/Myelography (NCHRT2W- MRC) derived from heavily 3D T2-weighted MR sequences (such as fast imaging with steady state acquisition, CISS, FIESTA, or sampling perfection with application optimized contrast using different flip angle evolutions) has been developed to overcome the above-mentioned limitations of CE- CTC/M and RC11-14. High contrast-to-noise ratio, multiplanar evaluation of volumetric data, and thin- section image acquisition are the main advantages of NCHRT2W-MRC5.
This paper will review the imaging diagnosis techniques and a comprehensive, state of the art comparison of the latest radiological advances and refinements, as well as a practical clinical approach in the two most important clinical CSF flow alterations: CSF rhinorrhea and intracranial hypotension syndrome.
METHODS & RESULTS
A comprehensive search in accordance with PRISMA guidelines (Preferred Reporting Items for Systematic Reviews and Meta-Analyses) using search terms of interest was completed through PubMed, Scopus, and reference lists from relevant articles to identify literature on sensitivities and accuracies of different imaging methods for localization and detection of CSF leaks. Search terms included were CSF leak imaging, CSF rhinorrhea imaging, spontaneous rhinorrhea and otorrhea, CSF otorrhea imaging, radioisotope cisternography, intrathecal Gd enhancement, MR cisternography, and intracranial hypotension imaging. These studies included meta-analyses, original prospective and retrospective papers, case reports, and others (technical notes, appraisals, commentaries, or editorials) published from 1995 until present. Reference lists of these articles and ongoing investigations in this area were also included. All papers had a Level of Evidence of 2 or 3, as no studies with higher Level of Evidence, such as randomized controlled trials, were identified.
A total of 857 papers were selected: CSF leaks, 661; IHS, 95; MR cisternography, 47; spontaneous rhinorrhea and otorrhea, 18; RC, 12; intrathecal Gd enhancement, 24. From these, only 143 were considered valid for the purpose of the revision. Meta-Analyses studies were 4; prospective studies were 16; retrospectives 25; short case series and case reports 41; reiews 31, and others 26.
RATIONALE AND BASIS FOR THE USE OF INTRATECHAL PARAMAGNETIC CONTRAST
Underlying mechanism of CSF enhancement by Gadoliniuni (Gd) on T1-weighted MR images rely on shortening the relaxations time of the water when a diluted paramagnetic contrast agent interacts with neighboring water protons (proton relaxation enhancement effect) 17-21. At lower concentrabonsof Gd, the main effect is on T1, while at higher concentrations the main effect is on T2, and paradoxical phenomena may appear on T1 (Fig. 1). The lower dosage of diluted Gd used (0.2-5 mL) is adequate for diagnostic subarachnoid enhancement on MRI in most clinical situations11-23. At these doses, CE-MRC provides excellent contrast and anatomic depiction of CSF-containing spaces and surrounding tissues.
Figure 1. Imaging of mechanism of CSF enhancement by Gd on T1-weighted images due to shortening the relaxation time of the water when different concentration of paramagnetic contrast agent are diluted, and subsequent paradoxical phenomena. Axial T1-weighted image after intracavitary Gd injection show giant cyst- like appearance of a craniopharyngioma with a supernatant hyperintense content and absence of signal in the subnatant gravitation-dependent fluid level. This is due to the paradoxical low signal caused by increased contrast concentration.
(Reproduced from Muñoz A et al77 with permission).
ETHICAL AND LEGAL QUESTIONS. SAFETY AND HAZARD CONSIDERATIONS
The safety of intratecht GBCA have been reported in several pilot studies in Europe6,9,10,14,25. Tolerance and dose limits of intrathecal Gd injection were established in animal model studies, and different Gd compounds or gadolinium-based contrast agents (GBCA) were compared in different experimental animal protocols26-28 both animals and humans. Its safety and tolerance have been well established in clinical trials in rhinorrhea/otorrhea and spinal CSF leaks, with a the low incidence of side effects6,8,10,21-26,28-33. Furthermore, CE-MRC seems to have a higher sensitivity in detecting pathology than their CE-CT corresponding proceriUreS23•34-37.
Animal studies have demonstrated that a high dose of intrathecal GBCAs, such as gadopentetate tlieghinft and gadodiamide, can result in signs of neurotoxicity such as gait disturbance, myoclonus, ataxia, tremors, and seizures37-39 both in animals and humans. These adverse events occur at doses equivalent to 5-15 umol per gram of brain tissue and are associated with histopathologic findings, including loss of oligodendroglia, astrocytic hypertrophy, and eosinophilia 18.40. Some case reports in humans have shown evidence of neurotoxicity after intrathecal injectionm4143. Most of these cases involved accidental overdoses, which resulted in signs and symptoms such as confusion, global aphasia, vomiting, stupor, rigidity, seizures, and hypertension.45. It was concluded that these changes and disturbance did not occur if the total injected dose was 15 mL (3.3 mmollg) 3 4. Direct intraventricular injection of Gd has been subject to trials only in animal models. In humans, only 3 patients had an inadvertent direct intraventricular high dose injection of Gd-DTPA, and in one of the patients with fatal outcome43.
Intrathecal use of GBCAs is not currently approved in many countries and it is not approved by the FDA in the US or EMA in the UE. Therefore, the clinical use of intrathecaUintraventricular GBCA are restricted to specific clinical scenarios and always performed when judged to be a better or the only alternative.
Health Systems organizations in some countries require that physicians opt for the so-called merciful use of drugs. That means that the team of doctors responsible for the care of a patient may use a drug that has been approved for other conditions as long as it is prescribed on an individual basis, the patient is never enrolled in a clinical trial, and helshe acknowledges after being informed that drug is not officially approved for the proposed study. Moreover, multiple other relative contraindications exist to performing contrast intrathecal injections, which must be taken into consideration before carry out any study such as RC, CE-CTC, or CE-MR. In particular,these include a history of seizures, a grossly bloody spinal tap, hematoma or infection at the puncture site, pregnancy, space-occupying intracranial process with increased intracranial pressure. evidence of bleeding disorder or coagulopathy, myelography performed within 1 wPPk, previous surgical procedure in anticipated puncture site, generalized septicemia, and a history of allergy or intolerance to iodinated contrast media and/or gadolinium-based MR contrast agents. Complications of intrathecal injection include postprocedural headache, infection, and minor bleedings.
The literature review was unableto identify any randomized controlled trials of GBCA, because of its off-label use, and all studies were observational with no control groups. Furthermore, because of the ethical constraints associated with administration of an off-label substance, it would be difficult to conduct randomized controlled trials to assess intrathecal GBCAs safety. According to the largest meta-analysis conducted, Patel et a146 reviewed 33 studies, none of them randomized controlled trials, that included a total of 1036 patients who had received intrathecal GBCAs, the overall rate of reported adverse events was 13%; although the rate of serious adverse events was not amenable to meta-analysis, serious events were noted in 10 patients. The authors concluded that intrathecal administration of GBCAs were not associated with serious neurotoxic adverse effects at doses lowerthan 1.0 mmol. The m ost commonly reported adverse effect associated with intrathecal injection of GBCA was a transient postural headache lasting less than 24 hours, occurring in 13% of cases, that could be confounded for postural headaches commonly seen after LP procedures, with a reported incidence of up to 49% in the literatuream, the discrepancy between the incidence of postural headaches in our analysis and the literature is likely due to an underreporting of minor symptoms associated with lumbar puncture given that adverse events after intrathecal administration ofGBCAs was notthe primaryfocus for most studies44. Few patients reported nausea, emesis, or fever. Serious adverse events reported in 10 patients included various symptoms relating to neurotoxicity, including ... seizures, dysarthria, ataxia, confusion, visual disturbances, vertigo, myoclonic jerks, aphasia, changes in extremity muscle tone, and mental status changes, i4. The authors also found a strongly statistically association between the seriousness of the adverse event and the dose of the intrathecally administered GBCAs. All minor adverse events were reported in patients who had received 1 mmol orless of GBCA intrathecally (mostwere 0.25 or 0.5 mmol), whereas the intrathecal dose for all reported serious adverse events was 2 mmol or greater (range, 2-10 mmol). All serious adverse events, but one, occurred acutely, with nine of 10 studies reporting adverse events within the first 6 hours after intrathecal GBCAexposure46.
Although not strictly an adverse event, it is consider the issue of increased central nervous system gadolinium chelates deposition within the human brain extravascular space after repeated intravenous administrationsu. In this issue, GBCA can be classified into linear and macrocyclic agents. Macrocyclic agents, such as naninutrol are more recent agents developed to decrease the potential side effects of GBCA46.49•51. There are several reports searching for the MRI brain changes and effects of the intrathecal injection of linear GBCA52.51. In term of CNS chronic aunts deposition the new macrocyclic agents have proved not to show these effects previously reported with linear agents, although its toxicity are also dose- dependent46. In particular with this regard, there are two prospective studies searching for the side clinical effects and CNS contrast deposition of gadobutrol at a dose of 0.5 mmol, which concluded that its intrathecal administration in doses of either 0.25 or 0.5 mmol was safe and without serious effects54,55 and that intrathecal macrocyclic GBCA administration was not associated with measurable T1 signal intensity changes in the globus pallidus and dentate nuclei, as an indicator of brain gadolinium deposition detectable by MRI52. For these reasons, currently there is a trend towards change in GBCA administration from the linear ones to the macrocyclic, not only in its intrathecal use but also in any administration route.
CURRENT MAIN INDICATIONS OF GADOLINIUM-ENHANCED MRI CISTERNOGRAPHY/MYELOGRAPHY
1. CRANIAL CSF LEAKS (CSF-RHINORRHEA AND OTORRHEA)
CSF leaks are extracranial egress of CSF into the adjacent paranasal sinus (rhinorrhea) or tympanomastoid cavity (otorrhea) due to an osteo dural defect involving skull base32. CSF fistulas can be classified as traumatic/postsurgical, spontaneous, or iatrogenic according to etiology. Traumatic cases are the commonest occurring in up to 30%56,57and up to 30% of skull base surgeries58. The most common sites were ethmoid roof, cribriform plate, and sphenoid sinus35. Traumatic CSF leaks often close spontaneously and may be treated conservatively. However, if persistent, the CSF leakage site may be a port of entry for bacteria, causing meningitis or other complications, and may need surgical repair59. Precise localization of the fistula before surgery increases the rate of successful repair and decreases the duration of the operative procedure. Although many non- radiologic tests and ancillary procedures may help to refine the diagnosis in patients who required surgical treatment, a definitive diagnosis depends on demonstration of the fistula site by imaging procedures. CSF leaks may also occur in patients without any known precipitating factor, so called spontaneous CSF leaks, comprising up to 40% of a CSF case series60. Spontaneous leaks are an increasing recognizable cause of CSF-leaks seen in obese, middle-aged women with sleep apnea and orthostatic headaches, who present with clinical features and imaging findings of increased intracranial pressure, similarly as those seen in patients with idiopathic intracranial hypertension (IIH), normal CSF, and without imaging evidence of mass lesion or hydrocephalus61.
Typical MR imaging findings include prominence of perioptic CSF spaces, posterior scleral flattening or protrusion of optic nerve head, prominent arachnoid granulations causing skull base erosions, empty sella and meningoencephalocele32. Ancillary radiologic findings associated with IIH include bilateral transverse sinus stenosis and low-lying cerebellar tonsils along with inferiorly displaced brainstem and cerebellum as in Chiari 1 malformation62. The proposed mechanism for the development of menin- goencephalocele and spontaneous leaks in IIH is due to impaired CSF absorption by arachnoid granulations causing fluctuating or persistent elevation in intracranial pressure leading to bony erosions which can facilitate herniation of meninges and brain tissue through the areas of least resistance10. There are also CSF leaks caused by congenital or acquired breaches of the osteodural barrier (congenital encephaloceles, primary empty sella syndrome, Stemberg’s canal, tumor erosion, etc.), that are often included within the spontaneous CSF leak group, and whose incidence has not been properly established63.
CSF-otorrhea is a particular form of base of the skull leaks resulting from abnormal communications between subarachnoid spaces and pneumatized parts of the temporal bone and sinonasal tract through osteo dural defects at the base of the skull32,56 and could be associated with rhinorrhea because CSF may leak through the Eustachian tube into the nasopharynx/nasal cavity64. The presence of temporal bone fracture along with air pockets in inner ear structures, including vestibule and semicircular canals, help to direct the imaging procedure workup.
Identification of glucose in nasal or ear secretions has been widely reported for the identification of CSF, given its availability, cost, and ease to perform. However, its sensitivity and specificity are low, since hyperglycemia, epithelial inflammation, bacterial infection or contamination with nasal mucus, blood and tears may alter its result65. Zapalac et al66 proposed a diagnostic algorithm for skull base CSF leaks in 2002 involving confirmation with beta-2 transferrin and that shoudl always be measured before any invasive procedure, so that excluding non specific causes of rhino-otorrhea.
The initial imaging techniques that can be used for preoperative assessment of the patients to determine the CSF-fistula site are firstly non- invasive, including high-resolution computed tomography (HRCT) and non-contrast enhanced MR cisternography (NCE-MRC). Imaging mini-invasive studies including RC, CE-CTC, and CE-MRC are utilized in selected cases. The choice of the imaging modality and its diagnostic accuracy depends on the local experience and expertise along with technical capabilities of the modality.
Radioisotope Cisternography
The technique consists of intrathecal injection of radiopharmaceutical radiotracers, either technetium (99mTc)- or indium (111In)- labeled Diethylenetriamine pentaacetic acid (DTPA), with periodic imaging. Usually acquisition of the images as standard protocol consists on imaging at 1, 2, 4, 24, and 48 hours postinjection. Imaging at multiple time points aids in the detection of intermittent or slow leaks45. Detection of the radiotracer activity in the sinonasal cavity or the nasopharynx indicates a positive study. However, RC is no loger an imaging procedure in the detection of the base of the skull CSF fistula and it is rarely performed now‑a‑days for the following reasons. Firt, active CSF leakage should be present at the time of study for accurate diagnosis. Second, intermittent CSF leaks are poorly demonstrated by Tc‑99m radionuclide study, although a prolonged cisternography could be performed with a radiotracer having a longer biological half‑life such as the Indium 111 diethylenetriamine pentaacetic acid and delayed imaging could be performed with this radionuclide for up to 72 h for diagnosis of intermittent CSF leak1.Third, the accuracy of RC is reported to be 70% in active leaks and much lower (i.e., about 28%, in inactive leaks, low-flow fistula or hair like communications).
Computed Tomography
Non-contrast HRCT of the base of the skull, specifically paranasal sinuses and the temporal bones, is usually the first line of image performed in patients with CSF leaks67. Nowadays multidetector CT scanners acquire isotropic, volumetric, thin submillimetre bone algorithm sections, allowing multiplanar reformations with excellent delineation of bony anatomy with unparalleled identification of any bony defect. The images can also be used for intraoperative navigation along with endoscopic views. Shorthcomings of HRCT are insufficient demonstration of the concomitant dural defect and to distinguish mucus secretions from the CSF collection within the air cells adjacent to normal areas of bony thinning/dehiscence in the skull base68, particularly in postoperative or posttraumatic states since high attenuation bone fragments, bone artifacts and anatomic bone detail makes the images difficult to evaluate, and at times inconclusive (fig. 2). Overall, the sensitivity of HRCT for CSF leaks varies between 84–95% with specificity between 57–100%69,70. Identification of a single defect in a patient with a positive correlation between the location of the bony defect and clinical symptoms obviates the need for further imaging.
Fig. 2 (A-E). CSF rhinorrhea following head trauma in a 35-year-old man. A, coronal thin-section CT scan reveals a defect in the roof of the sphenoid sinus (arrow) and opacification of the right sphenoid sinus. B, A coronal T1-weighted fat-saturated MR cisternogram obtained after the intrathecal administration of Gd-DTPA shows contrast leakage (arrow) extending from the cranial subarachnoid space into the right sphenoid sinus. C, after repair of the dural rupture, suspected CSF rhinorrhea recurred 1 week later and the patient underwent control MR cisternography. Images obtained in the first hour show probable leakage (arrow). D and E, Leakage becomes obvious in late images taken in the third and fifth hours (arrows). (Reproduced from Selcuk H et al10 with permission).
Non-contrast enhanced MR cisternography (NCE- MRC)
The principle of NCE-MRC cisternography is to demonstrate a contiguous fluid signal between the cisternal space and nasal sinus on heavily weighted T2 images. Hence, imaging during prone position is recommended. NCE-MRC exploits the relative high signal intensity of the CSF in subarachnoid spaces using heavily T2-weighted sequences with suppression of background signal from the soft tissue and cerebral parenchyma. The major disadvantage is the lack of bony detail. When positive, NC-MRC leakage is characterized by a hyperintense tract between the subarachnoid space and the sinonasal spaces32,71. Submillimeter acquisition and multiplanar reformats facilitate optimal evaluation of the skull base defects72. The reported sensitivity of NCE-MRC in the detection of a CSF leak is about 94%73.
Due to excellent soft tissue resolution, NC-MRC facilitates delineation of contents herniating through the bony defect and differentiates meningocele from an encephalocele with adjacent gliotic parenchyma74. NCE-MRC yields higher accuracy than HRCT for non-invasive diagnosis and localization of CSF fistula. As a result, NCE-MRC can be chosen as a first-line imaging technique because there is no ionizing radiation exposure or contrast medium administration, and this technique is non- invasive and has a high soft-tissue contrast without artifacts from bony elements35. However, in surgical cases non-contrast MR cisternography must be interpreted along with HRCT as MR imaging fails to provide optimal bony detail74,75. In more complicated situations such as in patients with positive results on a beta 2-transferrin test but no visualized CSF leak or suspicious leak on NCE-MRC, CE-MRC should be performed (fig. 3).
Fig 3 (A-C). Example of false positive NC-MRC and true positive CE-MRC. Coronal T2WI (A), coronal intrathecally CE- MR (B), and axial intrathecally CE-MR fat-suppressed T1WI (C) of a 52-year-old man with suspected CSF rhinorrheaof a 52-year-old man with suspected CSF rhinorrhea. NC-MRC falsely depicts a possible leakage at the frontal sinus roof (white arrow head), whereas CE-MRC correctly points to a surgery proven left ethmoid region defect (white arrows). (Reproduced from Ecin G et al71 with permission).
Contrast-enhanced CT cisternography
CE-CTC only elicits the site of leak in active cases and should be compared with HRCTdone priorto contrast instillation foroptimal evaluation ofcontrast leak. The ma in indication of C E-CTC is to assess CSF leaks in patients with multiple fractures and osseous defects, and those with a suspicious bony defect and dinically confirmed leaks32. A positive study is considered when there is contrast pooling throughout and down back to a bony defecto. The sensitivity of CE-CTC ranges between 85-92% in patients with active leak and 40% in those with inactive leakm. The main shortcomings of this technique are the low sensitivity in patients with inactive/intermittent leaks andobscurationofsmall leakdueto non-leakage of high viscosity contrast, adjacent to high-density bone70,74 (Fig. 4). Also, drawbacks of the CE-CTC procedure includes its mini-invasive nature, increased radiation dose due to multiple scans, inherent risks associated with a lumbar puncture and intrathecal instillation of iodinated contrast.
Fig 4. Spontaneous rhinorrhea, which turned out to be secondary to a base of the skull defect, whose CE-CTC was negative. Midsagittal T1-weighted fat-suppressed CE-MRC through the base of the skull shows contrast leakage in the sphenoidal sinus (arrow).
Fig. 5 (A-E). An 18-year-old man with recurrent meningitis after trauma. A, coronal high-resolution CT image demonstrates the bone defect in the left cribriform plate (arrow). B, Cerebrospinal fluid (CSF) leak from the left cribriform plate is nicely seen on the coronal three-dimensional constructive interference in steady state (3D-CISS) image (arrow). The size and relationship of the defect with neighboring structures and CSF leak (arrows) is clearly depicted on non-enhanced coronal (B), axial (D), and coronal (E) contrast-enhanced MR cisternography (CE-MRC) images. (Reproduced from O. Algin et al9 with permission).
Contrast-enhanced MR cisternography
CSF leaks are the most common clinical condition for CE-MRC is performed, particularly in spontaneous or posttraumatic/postsurgical rhinorrhea, outperforming CE-CTC. The sensitivity ranges from 92–100% in patients with active leaks and about 70% in patients with inactive leaks with a reputed specificity of 80%.
The main advantages CE-MRC are detection of intermittent CSF leaks (fig. 2 and 5), which can be attributed to its ability to perform delayed imaging up to 24 hours76, demonstration of low-flow fistula or hairlike communications, a depiction of meningoceles (fig. 6), and relative ease of assessment of leak compared to CE-CTC, attributable to the differentiation between the hyperintense contrast and adjacent bone10,21,66,69.
State of the art imaging in this setting introduces the so called CS T1 SPACE sequence, which offers a global 3D view of the entire skull base, meninges, and brain with high spatial detail and a global 3D view78 (fig. 7). This technique is particularly suitable as a problem-solving tool using macrocyclic GBCA in patients with high clinical suspicion of intermittent leaks. In cases of otorrhea CE-MRC has shown high promising (fig. 8). The single center longest CSF- otorrhea series, that involves 16 patients who underwent CE-MRC, reported an overall sensibility and specificity quite high and superior to HRCT (89.3 and 72%, respectively)30. The main shortcoming of CE-MRC is the lack of US and EU legal approval for the intrathecal administration of GBCA. Table 1 proposes a diagnostic algorithm for skull base CSF leaks.
Table 1: Diagnostic algorithm for skull base CSF leaks (Taken from Zapalac et al66 with modifications). HRCT: high resolution Computed Tomography; PNS: paranasal sinus; CE-MRC: contrast-enchanced MR-cisternography; NCE-MRC: non-CE-MRC.
Fig. 6 (A-C). Example of full MRI demonstration of the pathologic contents in complex postsurgical states. MR imaging of a craniofacial malformation (Crouzon syndrome) after surgical fronto-orbital advance. Two months after the procedure the patient experienced intermittent rhinorrhea. Midsagittal CE-MRC image (A) before surgery shows cranial deformity and enlargement of ventricles. No base of the skull defects are seen. Midsagittal (B) and coronal (C) CE- MRC images at the level of the third ventricle and after intrathecal Gd injection demonstrates a CSF leak into the left nasoethmoidal (thin arrow) space and an acquired meningoencephalocele through the anterior base of the skull (thick arrow). (Reproduced from Muñoz A et al31 with permission).
Fig. 7 (A-C). A 55-year-old woman (patient 1) who was readmitted for recurrent spontaneous left-sided CSF rhinorrhea and persistent slight headaches while sitting or standing after previous endoscopic sinus surgery. One year earlier, sealing of cribriform plate with TachoSil (Takeda Pharma) for suspected CSF leak had been performed. CT cisternography (soft tissue window level, W: 350; L: 50) is unremarkable, even in retrospect (A and B arrows). CS SPACE MR cisternography demonstrates a subtle CSF leak originating from the anterior rim of the left cribriform plate near foramen cecum (C and D, arrows) with a thin CSF collection extending to the anterior nasal cavity (arrowheads in C and E). The site correlated with the patient’s intraoperative findings. After surgical repair, the patient was free of CSF rhinorrhea. (Reproduced from Duman IE, et al78 with permission).
Fig. 8 (A-C). Spontaneous otorrhea and dizziness in a patient with CSF labyrinth fistula. A and B: axial and coronal CT scan through the left middle ear and osseous labyrinth show absence of osseous cochlear dysplasia (A) and bulbous appearance of the internal auditory canal (arrow in B) with no middle ear or mastoid cavity filling. C, T1-weighted fat-saturated CE-MRC image through the upper medulla oblongata shows abnormal filling of the left cochlear structure (arrow). D-F, T1-weighted fat-saturated CE-MRC images through the cerebellopontine cisterns. Axial (C) and spot view of the right side (D) show CSF filling up to the lamina cribrosa. However, further membranous labyrinth structures (semicircular canals) are filled on the left side (arrow in E) as well as cochlear duct (arrow in F). (Reproduced from Muñoz A et al31 with permission).
2. SPONTANEOUS INTRACRANIAL HYPOTENSION
SIH is caused by spinal CSF leaks, through small spinal dural tears, leaking meningeal diverticula, and CSF-venous fistulas, resulting in continued loss of CSF volume, leading to CSF hipovolemia, thus promoting multiple debilitating clinical manifestations79. It is pathogenetically separated from that of LP, and from postoperative CSF loss80. Also, CSF leaks due to penetrating dural injury from major traumatic events or iatrogenic cause are not considered to represent SIH and are classified separately81. Moreover, their clinical course and management differ.
The diagnosis of SIH remains difficult due to the various clinical manifestations, some of which are nonspecific and easily to be neglected81,82. Major risk factors for SIH include disc degenerative diseases and underlying connective tissue disorder, such as Marfan syndrome or Ehlers-Danlos syndrome, hyperflexible joints neurofibromatosis, and Lehman syndrome, which predisposes to weakness of the spinal dura mater such as dura meningeal diverticula and dural tears83. Besides, patients with these genetic connective tissue disorders, are at higher risk of spontaneous spinal CSF leaks than the general population84,85.
For he purpose of this review from here on we will refer specifically to cases of spontaneous SIH. Differential diagnosis of SIH with other syndromes presenting with orthostatic headache is crucial, but it is beyond the scope of this review. SIH can occur at any age, although most patients are middle-aged and there is a female preponderance (female: male ratio of 1.5: 1)86 with an estimated annual incidence of 5/100,00082. Trauma, ranging from motor vehicle accidents to minor incidents such as sporting injuries, tumble, or even sneezing, has been implicated; these potentially rupture meningeal diverticula or cause tears in the dural nerve sheath87.
Proposed diagnostic criteria for SIH include clinical and imaging findings that suggest intracranial hypotension, or directly demonstrate an active spinal CSF leak34,88-90. According to the International Classification of Headache Disorders, third edition, SIH is diagnosed when headache has developed spontaneously and in temporal relation to a CSF leak (evident on imaging) and/or CSF hypotension (lumbar puncture opening pressure
<60mmCSF80. These criteria are probably the most widely accepted, but do not capture the full spectrum of patients with SIH. In fact, SIH is actually a misnomer, as the majority of patients exhibit opening CSF pressures within the normal range91,92. The true pathophysiology is therefore more appropriately described as reduced intracranial CSF volume rather than CSF hypotension93,94. Moreover, low opening pressure is, however, an unreliable marker of the disorder because it has been reported in only a third of patients with confirmed spontaneous intracranial hypotension95, and could have led clinicians to exclude this diagnosis in patients with normal pressure. This is why diagnostic criteria have changed significantly throughout the last few decades, and alternative diagnostic criteria have been proposed96,97. These factors have probably contributed to the current uncertainty on how to reliably diagnose SIH and effectively treat these patients79. For this reason SIH was misdiagnosed in up to 94% of individuals, most commonly as migraine, meningitis, psychological disorder, or even malingering108.
The reasons why these patients with SIH have normal CSF opening pressure on LP might be related to the inadequate methods of measurement or to the actual absence of a low CSF pressure state. LP opening pressure is a snapshot method of measurement, it does not reflect the intracranial pressure in the upright position, and neither offer any information regarding the CSF dynamics during a postural change. The correlation between SIH and connective tissue disorders supports the hypothesis of a dural compliance disorder as the main cause for this syndrome98,99. Although LP has a good sensitivity (67%) and can support the diagnosis of SIH, a normal opening pressure does not exclude this disorder; therefore, the diagnosis of SIH cannot be excluded in patients who do not present with all the typical features of this disorder79.
The clinical manifestations of SIH are thought to result from increased efflux of CSF in the upright position, leading to traction on pain- sensitive fibres within the dura mater. Despite the variability and the great diversity of signs and symptoms orthostatic headache, particularly from occipital location, subsiding after lying down and worsening with Valsalva maneuvers, is the most common and hallmark symptom, affecting patients during their most active hours making SIH syndrome a debilitating chronic disease79. Nonetheless, headeache in SIH might be gradual or thunderclap in onset, less or more positional over time, even intermittent, and with no relationship with position, or become worse when lying down, and its intensity is variable90. Also, the orthostatic headache, once believed to be an essential characteristic of SIH, is not invariably present and SIH should not be excluded based on the absence of orthostatic headache79.
Other signs and symptoms such as posterior neck or interscapular pain, horizontal diplopia caused by unilateral or bilateral cranial nerve VI palsy, nausea, emesis, radicular upper extremity symptoms, dizziness, blurred vision, change in hearing, or obtundation may also be present79 as a result of a decrease in CSF volume and sinking of the brain, but, as mentioned, patients with atypical clinical signs and normal CSF pressure may also be found85,100. Cochlear vestibular manifestations (e.g., tinnitus, hearing change, and dizziness) are often seen101 and may be related to traction of the VIII cranial nerve, but an alternative and more plausible mechanism could be the alteration of pressure in the perilymphatic/endolymphatic inner ear fluid by alteration of the pressure gradient between CSF and perilymphatic fluid that are in contact at the level of the acoustic aqueduct. This would cause an endolymphatic hydrops such as Menière’s syndrome102. Other atypical presentation of SIH, seen in older patients with SIH with a longer symptom duration, are memory deficits, dementia with frontotemporal features, parkinsonism, and ataxia103. Tanaka et al found that more females and patients under 40 years of age reported acute onset and severe headache, whereas more males and patients over 40 presented with subdural hematomas and reported longer durations of symptoms before presentation104. Whether these patterns reflect biologic features of SIH or reflect presentation bias trends is uncertain91. Due to the woefully inadequate awareness of it, the ratio of initial misdiagnosis and missed diagnosis is pretty high, and it also contributes to the long mean time (13 months) from symptom onset to diagnosis105. Finally, in clinical grounds, despite not typically being considered a serious or life-threatening disorder, spontaneous SIH can cause considerable morbidity, result in substantial long-term disability, and (in rare cases) lead to decreased consciousness and coma93,106,107. The mechanisms proposed in published work to account for this presentation include transtentorial herniation with compression of the diencephalon, and traction on cerebral sinuses resulting in deep venous thrombosis93. As a result of low CSF volume—sagging of the brain—tensión on the cranial nerves and the dura mater occurs, particularly is in the posterior fossa, which is especially tension-sensitive108.
A previous common misconception is that skull base CSF leaks cause intracranial hypotension; this is not generally the case91. Normally, when upright, gravity causes a gradient of increasing CSF pressure as one moves caudally along the spinal axis, with the result that intracranial pressure is slightly lower than atmospheric pressure in this position109. This gradient disappears in the recumbent position. As a result of this physiology, spinal CSF leaks cause orthostatic headaches while leaks at the level of the skull base do not. Rather, skull base defects tend to leak CSF when patients are bent over, leading to CSF rhinorrhea or otorrhea, not orthostatic headaches. This lack of association between SIH and skull base CSF leaks has been confirmed in a large series of patients with SIH110.
In non-syndromic patients with SIH, leaks are predominantly, if not exclusively, due to mechanical tears, CVFs, and leaking nerve root sleeves/meningeal diverticula and, according to that, they could be classified in four types111.
Type 1 leak, or dural tear type, is caused by degenerative disc disease caused by an osteo discogenic microspur (eg, a calcified disc protrusión, osteophyte or vertebral outgrowth) that pierce the dura like an ice pick, penetrating the thecal sac, and creating a mechanical tear in the ventral dura111,112; nonetheless, conspicuous disc protrusions or large spinal nerve root cysts do not always correlate with the level of CSF leakage93 (Fig 9). They are far more commonly located within the thoracic spine or lower cervical spine113,114. The propensity for these locations may be due to the close apposition of the dura to the posterior aspect of the thoracic vertebral bodies and discs as a result of the normal thoracic curvature, combined with a higher rate of calcification of thoracic disc protrusions that can erode the dura to a greater degree than non- calcified disks91,115,116,117. Patients with these ventral dural punctures are well-recognized as representing a large segment of patients with SIH, ranging from 26.6 to 48% of cases86,91. All patients with type 1 show a typical ventral hole in the thecal sac on digital subtraction myelogram (DSM) or dynamic CT-myelography (DCTM) 86,111,115 (fig. 10). Type 2 leak, or meningeal diverticulum type, is due to a more lateral tear in the dura due to leaking spinal nerve root diverticula/dural ectasia.
These leaks are thought to arise from the base of the nerve root relating to a pre-existing thinned or dehiscent area of the dura in the axillary sleeve region of a nerve root. This herniated arachnoid may rupture, producing a fast CSF leak, or CSF may seep slowly from the margins of the dehiscence. Most commonly occur within the thoracic or upper lumbar spine and can have varied morphologies, in some cases solitary and in others complex and multilobulated117, (fig. 9). Its incidence ranges from 13 to 48% of cases86,112. Nonetheless, diverticula are common, and one study found that the number and configurations of diverticula are similar between patients without and with CSF leak117. Therefore, patients with SIH may have leakage from a particular spinal meningeal diverticula among many others that turn out to be common incidental findings117 (Fig. 11). Also, periradicular leaks may not necessarily represent the precise site of a CSF leak and lumbar puncture may cause an iatrogenic CSF leak118.
Fig. 9. Examples of the three types of CSF leak. Type 1: a ventrally located dural slit due to an osteo discogenic microspur (calcified disc protrusion or osteophyte) penetrating the thecal sac. CSF leaks into the epidural space. Type 2: a leaking spinal nerve root cyst. Type 3: a direct CSF-venous fistula. (Reproduced from Dobrocky T et al. Spontaneous intracranial hypotension: searching for the CSF leak. The Lancet Neurology 2002, with permission).
Fig. 10 (A-D). Middle-aged patient with sudden onset of orthostatic headache. A, heavily T2 weighted sagittal MRI with visible cerebrospinal fluid in the ventral epidural space, and the dura mater (arrow). B-D, dynamic myelography with contrast medium leaking into the ventral epidural space (arrow). E, postmyelography CT demonstrating a ventral microspur (arrow) at the corresponding level leading to a dural breach. (Reproduced from Piechowiak, EI et al 159, taken with permission).
Type 3 leak, or CVF, were discovered by Schievink et al119 and provided an extraordinary step forward in the care of patients with SIH, since it is a unique type of CSF leak because it is not associated with an extradural CSF collection110,121 (fig. 9). CVF are direct CSF-venous fistulas resulting from direct communications between the perineural subarachnoid space and surrounding veins. This aberrant connection allows unregulated loss of CSF back to the circulatory system120,121. The reason for this, whether related to meningeal fragility or juxtaposition of CSF to the veins, remains speculative82. Most CVFs occur between T7 and T12 and are associated with a meningeal diverticulum in 82% cases112 with the fistulous connection commonly originating from the diverticulum itself121. CSF- venous fistulas can also allow leakage into the epidural space in the absence of a dural tear122.
Farb et al proposed112 that an embryonic pseudomeningocele, masquerading as a diverticulum, first insinuates into the venous system and heals, aberrantly creating a 1-way CVF, which we categorize as type 3 CSF leak. This postulation also accounts for these CVFs being commonly but not invariably seen next to what appears to be a diverticulum. Its incidence is low, roughly 2,5%86. Although its true incidence is not well established and probably higher than previously believed because tailored radiologic diagnosis is in progress121. Most commonly occur along thoracic nerve roots. Such fistulas should be considered as a potential etiology for SIH in patients where a CSF leak cannot be identified91. Surgical ligation of a CVF.
Type 4 leak is the least common form of CSF leak and it results from a distal nerve root sleeve leak that does not fistulize into the venous system but rather tracks into and dissipates into the adjacent facial planes112. Type 1 and 2 leaks typically lead to CSF effusion from the intrathecal space to the epidural compartment, resulting in a spinal longitudinal extradural CSF collection (SLEC)125, and indicates a high-flow leak, typically type 1 or 2, and the need for an imaging method with good temporal resolution93, whereas type 3 and 4 leaks typically do not result in SLEC82.
Fig. 11. Plain T2-weighted fat-suppressed spine MR images in a female patient with HIS and multiple meningeal diverticula. Coronal thoracic and upper lumbar spine reconstructed CISS images show multiple spinal diverticula.
IMAGING
On imaging grounds, although routine contrast-enhanced brain MRI protocols are sufficient to evaluate for intracranial features of SIH, specialized noncontrast spine MRI protocols designed to detect extradural CSF can obviate conventional invasive myelography and aid in planning further invasive tailored imaging tests for the workup of SIH11,126. Once a leak or fistula is suspected on the basis of clinical features and/or non-invasive imaging, its location may be investigated by dynamic myelography, as a first step on mini-invasive procedures. If not, other most sopyisticated mini-invasive procedures such as DCTM or CE-MRM should be performed.
Brain
Many of the clinical and imaging manifestations of SIH can be explained by the Monroe-Kellie doctrine127. This states that within a mature intact skull the sum of the intracranial volumes of brain, blood, and CSF is a constant. When CSF hipovolemia occurs one of the other two constituents must be compensated for by an increase in one or both of the others. As the volume of the brain cannot increase, blood volume increases, primarily within the more compliant venous circulation. Consequently, several abnormalities might be observed on brain imaging of patients with SIH. These abnormalities generally result from intracranial CSF loss and a compensatory increase in the blood compartment, resulting in hyperaemia93.
Cranial CT
Although brain CT does not provide as many cardinal features as MRI, it is prior to more definitive imaging in the outpatients or emergency department and it is a helpful initial diagnostic tool for its non-invasive diagnosis90. Brain CT is often unremarkable in the context of SIH and is generally less useful than cerebral MRI90, but may show some subarachnoid hemorrhage85,128,129 or pseudo-subarachnoid haemorrhage secondary to brain sagging, since a reduced CSF volume efface the basal cisterns and Sylvian fissures, causing prominence of relatively hyperattenuating arteries and veins130 (fig 12).
Fig. 12 (A and F). Nonenhanced CT scan. A: Subdural hematomas (arrowheads) with minimal mass effect. F: Pseudo-subarachnoid haemorrhage. Notice increased attenuation in tentorium and cisterns/sylvian fissures (arrow). (Reproduced from Ferrante E et al128 with permission).
Cranial MRI
In the initial evaluation of undifferentiated headaches, brain MRI is often performed without contrast, but in suspected cases of SIH, contrast administration is essential91,94,131. On head contrast- enhanced MRI, diffuse regular pachymeningeal enhancement in both the supratentorial and infratentorial spaces is the most common imaging finding85,91,132 (fig. 13A), underlying the pathological substrate for this enhancement. Because the leptomeninges have a blood-barrier and pachymeninges do not, the latter are enhanced with Gd. This meningeal enhancement may be associated with additional imaging abnormalities including subdural fluid collections, occurring in about 50%85. Dural thickening and hyperintensity can also be shown on FLAIR sequences but it can be difficult to distinguish from adjacent subdural fluid collections on this sequence so the use of contrast medium is considered essential132,133. Several rare conditions can have a similar meningeal appearance on MR imaging, such as idiopathic hypertrophic pachymeningitis, Immunoglobulin 4.
As stated, volume may also be replace with subdural collections, either hygromas or hematoma, often bilaterally, and result from a decrease in the CSF volume within the fixed volume of the skull, leading to passive transudation of fluid out of the intravascular spaces in order to compensate for this volume loss. They are likely caused by tearing of bridging veins or bleeding from enlarged veins in the subdural zone89,91,12.
Bilateral hygromas or subdural hematomas secondary to SIH are important to exclude from other clínica settings and proper etiology must be identified because subdural fluid collections will not resolve until there is successful treatment of the underlying SIH (fig. 13 B-D). Moreover, if the collections are drained, they will recur due to the underlying abnormal CSF dynamics140. Therapy should be focused on finding and treating the leak rather than drainage of the collections139.
Venous distensión, secondary to small thin-walled dilated blood vessels in the subdural space, is another specific indicator of decreased intracranial volume141. It is a feature of blood volume compensation as a consequence of CSF volume loss and is present in 75 to 93% of cases96,141. It is reported that venous sinus thrombosis may be more prevalent in patients with SIH compared with general population for several reasons139. Also, venous distension is the first sign to reverse after successful treatment of SIH89.
Low-lying cerebellar tonsils or cerebellar tonsillar descent through the foramen magnum is usually seen in cases of very severe mid-brain sagging. However, it should be interpreted with caution when other findings of brain sagging are absent, since this sign is also present in Chiari malformation, a congenital condition that can be treated by posterior fossa decompression. Thus, it is especially important to distinguish this sign carefully, particularly as it can also be unreliable93.108. Conversely, when cerebellar tonsillar ectopia is present in patients with headaches, the imaging should be scrutinized for other signs of brain sagging before the diagnosis of Chiari I is madem. Moreover, individuals with Chiari malformation have other very important imaging features, are usually diagnosed at a much younger age, and present with different clinical symptoms compared with patients with spontaneous intracranial hypotension93.105.1
Typical findings of a leak are contained in the mnemonic SEEPS (subdural fluid collections, enhancement of the meninges, engorgement of venous structures, pituitary hyperemia, sagging of the brain)and are highly suggestive ofthe presence of a leak45.97. Superficial siderosis may occurs as up as 50% of patients with chronic spinal CSF leaks and SI HI42, as a result of repeated episodes of chronic subarachnoid11,5n. laagg from friable blood vessels at the margins of spinal chat defects and typically related to recurrent trauma from a spiculated osteophyte or disk protrust(50,143 or because of tractions of the cerebellum and/or =mkt veins on the skull base, leading to microhemorrhages and hemosiderin deposition144, causing hemosiderin deposition on the surface of the spinal cord, brainstem and cerebellum. This appears as fi near bvgainten sitv, mainly on the infratentorial brain pial surfaces, on susceptibility-weighted imaging or Tr-weighted gradient echo imaging (fig. 13 E). Approximately 50% of patients with posterior fossa superficial siderosis have a spinal extradural CSF collection, indicating a CSF leak141,145,146. Dural leaks causing recurrent subarachnoid bleedings can represent the single underlying cause for the superficial siderosis. The accumulation of this neurotoxic iron leads to progressive cell damage and neurological deficits147.
Fig. 13. Brain MRI features of HIS in a 56/y old female. A, coronal postcontrast T1-w image shows diffuse regular pachymeningeal enhancement in both the supratentorial and infratentorial spaces. B and C, axial FLAIR (B) and T2-W
(B) images through the lateral ventricles showing bilateral subdural collections. D, axial post contrast T1-weighted image at the same level shows diffuse meningeal enhancement. E, axial susceptibility-weighted image (SWI) through the brainstem and cerebellum shows preferential hypointense signal throughout the subpial surface of cerebellar hemispheres and medulla oblongata consisting on chronic hemosiderotic deposits.
Engorgement of the pituitary gland (8–11 mm), secondary to venous congestion, causes enlargement of the gland with a convex superior margin, occurs in 38% patients with SIH133, but is an unreliable sign of the condition, since gland size varies according to age and sex or an underlying infiltrative process, such as hypophysitis or adenoma, and care must be taken not to confuse this sign with physiologic enlargement as can be seen with pregnancy85. Moreover, hyperprolactinemia can also occur in SIH and is associated with brain sagging, possibly indicating a stalk-effect as the mechanism133.
Other MRI finding of SIH such as reduced optic nerve sheath diameter and diffuse calvarial hyperostosis are reported unreliable signs of SIH síndrome89.
The number of brain imaging signs required for diagnosis of spontaneous intracranial hypotension has not been clearly defined, and reliable cutoff values for quantitative signs are absent, although smooth, generalised (i.e., evenly spread) dural enhancement is quite typical, no single imaging sign is specific for spontaneous intracranial hypotension. For the purpose of triagering patients suspected of SIH in whom brain MR imaging is suggestive and may benefit from more invasive or expensive myelographic examinations and subsequent targeted therapy, a radiological scoring system that integrates the 6 most reliable brain MRI findings has been proposed131.
Pachymeningeal contrast enhancement, engorgement of the venous sinuses and effacement of the suprasellar cistern of 4.0 mm or less were shown to be the most important discriminating features between SIH patients and normal controls and weighted with 2 points each142. However, dural enhancement—the most common sign— was found to decrease in prevalence with time after symptom onset148. This study suggests that the sensitivity of brain imaging may decrease in patients who have been leaking CSF for longer periods of time, perhaps as a result of compensatory physiologic changes. Consequently, it is possible that patients with chronic CSF leaks may exhibit different physiology, imaging, and treatment responses than those with acute leak106. Finally, it has been estimated that up to 20% of patients with SIH have a normal brain MRI120,149
RADIONUCLIDE CISTERNOGRAPHY
RC, once used more extensively for spinal CSF leak localization, now is an elective initial indirect imaging test used in the evaluation of suspected SIH and, as it has had happened in the diagnosis of CSF-rhinorrhea and otorrhea, is rarely performed nowadays, and is increasingly considered obsolete, because it does not show the precise localization of a leak and its inherent of poor spatial resolution and inferior anatomical delineations, its invasiveness, requering LP, and radiation exposure97,105. The literature pertaining to the use of this technique in patients with SIH is quite limited and RC, in general, is infrequently performed compared to anatomical imaging studies7,91,150,151. Moreover, the greatest disadvantages of any mini-invasive procedure that require LP, apart from the complexity of the procedure, are iatrogenic CSF leaks152 and worsening symptoms153 caused by lumbar puncture.
Nevertheless, RC can be used when initial brain and spine MR imaging are negative for signs of SIH in a patient with equivocal symptoms of SIH síndrome and may be helpful in this instance to determine whether to proceed to further invasive testing.
In a normal nuclear medicine cisternogram, there is complete coverage of the cerebral convexities 24 h after intrathecal tracer injection in the lumbar spine. Incomplete convexity coverage at 24 h suggests impairment of CSF flow dynamics, as can be seen in SIH. Other potential findings on cisternography which may suggest any CSF leak include early visualization of soft tissues and the bladder. If the cisternography is positive, the patient will typically undergo lateral decubitus DSM or CTM to assess for a possible SLEC leak82.
CSF PET with 68Ga-DOTA, is a novel fast approach of RC for verification, though not localization, of spinal CSF leaks which hold promise for its high sensitivity and specificity, and it has the potential of the imaging methods to reduce the burden to the patient who eventually undergo other localizing invasive methods154. Although further prospective studies are needed to be validated and it is also an expensive invasive method.
SPINE IMAGING
In the upright position, hydrostatic pressure in the spine is positive relative to atmospheric pressure, and pressure within the intracranial compartment is slightly negative with respect to atmospheric pressure109. Therefore, the spine represents the anatomic source of most symptomatic CSF leaks and venous fistulas, such that investigation of the source of SIH should primarily focus on targeted imaging of the spine rather than the skull base, as mentioned earlier, because there is no causal association between SIH and cranial cerebrospinal fluid leaks110,126. It is important to take in mind that not every patient with orthostatic headache should be subjected to multiple spine imaging tests particularly invasives, because they have the potential for adverse reactions (to intrathecal contrast), may entail high radiation exposure, and are a financial burden on the health-care system156.
Identification and precise localisation of a CSF leak is the main goal. Although most leaks are reported in the thoracic spine and at the cervicothoracic junction, the leak can occur anywhere from the skull base to the sacrum. Therefore, when the spine is imaged the entirety of the spinal canal must be scrutinised for a dural breach that might be only the size of a pin93. The main usefulness of a spinal MRI is to identify an area of in the epidural space, suggestive of the site of CSF leak85,139. Whole spinal MRI from the cranial cervical junction to the sacrum with both T1 and T2 fat suppression sequences is a safe, non-invasive, and sensitive technique to identify spinal CSF leaks. They should be performed as a first-line clinical investigation whenever possible. Invasive imaging intrathecal-enhanced techniques that require LP should be reserved for patients without identified leak on spinal MRI, who have failed to respond to two or three epidural blood patches (EBPs) performed blindly and in whom a treatment targeted (EBP or surgery) at the site of a leak is being considered85.
Plain and iv contrast enhanced T1-weighted spinal MRI
When SIH is suspected, plain intravenous enhanced MRI of the entire spine is usually performed as a first-line clinical investigation. These procedures can support a diagnosis of SIH, but only rarely shows the location of the CSF leak Moreover, plain spinal MRI may yield false-negative results because a collapse of the dural sac and epidural CSF accumulations are easily missed, yet is still an excellent method of demonstrating the extent of ventral spinal CSF leaks90. Notice that as there are patients with SIH syndrome and no brain radiological features but positive of radiological spinal features, additional MRI of the brain head should be performed124.
Spine imaging aims to classify and localise the site of CSF leakage as either i) a ventral dural leak; ii) a leaking spinal nerve root diverticulum, or iii) a direct CSF-venous fistula (fig. 9, 10,14, 15 ,16, and 17). Precisely locating the site of CSF leakage is fundamental to successful treatment, which includes a targeted EBP and surgical closure when conservative measures do not provide long-term relief93. Plain and iv contrast enhanced T1-weighted fat suppressed spinal MRI may show abnormalities in 60-80% of patients102,124 and may demonstrate cervical pachymeningeal enhancement, non- compressive SLEC, fluid collection in soft tissues near the C1 and C2 vertebrae (it could be sometimes a CSF leak false localization sign), meningeal diverticula, dilated nerve root sleeves, engorgement of epidural venous plexus and SLECs, although it not always pinpoints the site oft he leak. SLECs are mostly associated with nerve root sleeve leaks, may be of variable size, with some producing high-flow leaks, and others producing quite subtle leaks. CSF-venous fistulas are notable negative in this regard because they usually do not result in pooling of fluid in the epidural space, and therefore may be difficult to detect on plain spine MRI.
Non-contrast high-resolution T2-weighted MR myelography (NCHRT2W-MRM)
Before proceeding with any invasive spinal procedure the new gold standard sequence of spine MR imaging is the NCHRT2W-MRM of the whole spine (3D fast spin-echo HT2W) for detecting CSF leaks without lumbar puncture and radiation exposure. Previous studies12 have shown that standard T2W-MRM was comparable to CE-CTC in diagnosing CSF leaks and is used to guide targeted EBP. Heavily T2-weighted MR myelography sequences are based on 3D isotropic steady-state free precession sequences (such as CISS or FIESTA17.12,14,155. which allow multiple plane reformats and are particularly helpful in demonstrating SLEC and in pinpointing the site of the breach (fig. 14). These sequences enhance CSF signals by suppressing the adjacent background signal. This new standard protocol overcomes the shortcomings of previous MRI protocols comprising 2D sequences that were sufficient to identify a dorsal or ventral epidural fluid collection, but CSF leaking laterally via a perineural cyst or lateral Mai defect may not be readily detected on those previous spine MRI protocols. In addition, the 3D sequence allows for the reconstruction of image data using various techniques, induding maximizing or minimizing CSF signal (MIP and MPR respectively tools). Therefore, NCHRT2W-MRM of the whole spine is warranted to evaluate not only the epidural space but also the paraspinal soft tissues for the presence of extradural CSF. Ancillary MR finding apart from epidural fluid collections includes distention of the spinal epidural veins, detected as prominent signal-intensity flow voids in the anterolateral epidural space with consequent collapsed asa, sac, appearing as a hexagon-like contour in the axial plane, seen in f 75%— 100% of cases156.
Fig. 14 (A-C). Central canal-type CSF leak. Whole spine sagittal 3D heavily T2-weighted MR myelography demonstrated diffuse CSF leak, from cervical to lumbar level (A) in a 45-year-old female patient. Axial image of heavily T2-weighted MR myelography revealed CSF leak at the epidural space in the central canal and the right neural foramen, at C7/T1 level (B) and revealed posterior epidural CSF leak at the T4/5 level (C). (Reproduced from Kim BR et al13 with permission)
Author Area
Have an article to submit?
Submission Guidelines
Submit a manuscript
Become a member