Home > Medical Research Archives > Issue 149 > Activin Stimulates Follicle Formation and Activation and Modulates Steroidogenesis in Fetal Bovine Ovarian Tissue in Vitro1
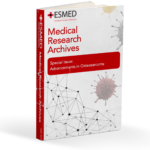
Published in the Medical Research Archives
Aug 2023 Issue
Activin Stimulates Follicle Formation and Activation and Modulates Steroidogenesis in Fetal Bovine Ovarian Tissue in Vitro1
Published on Aug 17, 2023
DOI
Abstract
The earliest stages of ovarian follicular development, follicle formation and development of the capacity to activate, are critical to the size of the ovarian reserve. In primates and most domestic animals, these stages occur during fetal life and thus, are difficult to study. Our laboratory developed methods in vitro to elucidate the regulation of these early stages in fetal ovarian tissue of cattle, an excellent model for human ovarian development. The current experiments were designed to determine if fetal bovine ovaries have activin A and its receptors and if activin A regulates follicular formation and/or follicle activation to begin growth in pieces of fetal ovarian cortex. During early to mid-gestation follicles first form in the ovarian cortex when oocytes are enclosed in a single layer of flattened granulosa cells. Activin increased the total number of follicles by 2-fold and the number of primary (activated) follicles by 5-fold in cultured ovarian cortical pieces, compared to control medium. During mid to late gestation, after follicles have formed, activin decreased primordial follicle numbers and increased primary follicles about 4-fold, indicating that activin stimulated primordial follicles to become growing, primary follicles. Our previous experiments showed that fetal ovaries produce ovarian steroids, especially just before and during early to mid-gestation (when follicles are forming), and that estradiol and progesterone inhibit follicle formation and activation. In control and gonadotropin-treated ovarian pieces, activin A increased progesterone, but decreased androstenedione and estradiol accumulation in the culture medium, suggesting that the effects of activin A are achieved, at least in part, by its modulating effects on ovarian steroidogenesis. Messenger RNA for activin and its type II receptors was detected in fetal bovine ovaries, with ACVR2A receptor mRNA more abundant than ACVR2B. Immunohistochemistry localized mRNA for activin A and ACVR2A to germ cells and granulosa cells at all stages and to theca cells of early secondary follicles. Staining for ACVR2B was weaker and mostly confined to germ calls. Taken together, these results strongly suggest that activin A of fetal ovarian origin plays a role in the establishment of the follicular reserve that is so important for normal female fertility.
Author info
Introduction
The goal of this study was to test the hypothesis that activin A regulates the formation of ovarian follicles and their activation to begin growth, using fetal bovine tissue in vitro as the experimental system. Activins are members of the transforming growth factor beta (TGF13) superfamily. They are homo- or heterodimers of the beta subunit of inhibin, which is present in two forms, A and B, giving rise to activin A, B, or AB, with activin A being the most abundant isoform (reviewed in 2). Activins signal through type I (ACVRA1and ACVR1B) and type II (ACVR2A and ACVR2B) receptors (reviewed int2). They are involved in many biological processes, including ovarian function, and there is evidence for roles for activins in early folliculogenesis512. Activin subunits and receptors have been detected in mammalian ovaries and activin is believed to be involved in a number of ovarian functions, including survival of germ cells, formation, activation and growth of follicles, and steroidogenesis (reviewed ins). To our knowledge, nothing is known about activins potential role in early follicular development in cattle, an excellent animal model for human ovarian development and functions. The goal of the current experiments was to determine if activin A plays a role in formation, development of capacity to activate, and activation of bovine follicles and/or in the regulation of steroid production by bovine fetal ovaries. Follicle formation and activation are the earliest stages of ovarian follicular development and as such they are critical for female fertility since they establish and regulate the size of the follicular reserve.
These follicular stages have been studied primarily in rodents where follicles form fairly synchronously a few days after birth, followed shortly by the activation of a subset of primordial follicles to begin growth and become primary follicles•. Our laboratory has developed methods for studying early follicular stages in cattle. In cattle, as in women, the early follicular stages occur during fetal life, making them difficult to study. The current experiments are focused on two periods of bovine follicular development. The first period is from about day 90 to about day 140 of the 280-day bovine gestation (i.e., early to mid-gestation). During this period, oocytes are enclosed within a single layer of flattened granulosa cells to form primordial follicles and follicles gain the capacity to activatelw. During the second period, after about day 140 of gestation, activation of a few primordial follicles begins and produces the first primary (growing) follicles. During activation the oocyte begins to grow and the granulosa cells become cuboidal. The current experiments were focused on determining whether activin plays a role in the critical developmental events that occur in early to mid-gestation (day 90-140) and in the period after day 140 (mid to late gestation). In cattle, as mentioned above, follicles begin to form about day 90 of gestation (gestation -280 days), but activated, primary follicles are not observed until about day 1401&17. Thus, there is about a 50-day period when newly formed follicles do not activate in vivo. When small pieces of ovarian cortex (the site of follicle formation) were isolated during this period (day 90-140; early to mid-gestation) and cultured for 10 days, the tissue remained healthy and follicle numbers and types did not change. If cortical pieces were cultured for 2 days with insulin there was also no change, but if the culture period was extended to 10 days, additional follicles formed and primary follicles were observed. We concluded that newly formed follicles do not have the capacity to activate in vivo, but that they could acquire that capacity in the presence of insulin in vitro^.
Adding exogenous estradiol or progesterone to culture medium with insulin inhibited both follicle formation and development of the capacity to activate^. This finding is consistent with the effects of steroids on follicle formation in rodents . These effects appear to be stage- specific since estradiol did not inhibit activation of cortical pieces from older bovine fetuses, after primordial follicles have developed the capacity to activate in vivo20. Fetal ovaries synthesize steroid hormones early in gestation, but steroid production declines just before and during the period of follicle formation^. When we increased endogenous steroid production by cortical pieces (day 90-140) in vitro with hormonal treatments, follicle formation and activation were inhibited21. Together, these findings point to insulin and fetal ovarian steroids as regulators of follicle formation and the capacity to activate in cattle, but it seems doubtful that they are the only regulators. Because activin A has been implicated in the regulation of later stages of follicular development, we hypothesized that activin A may also be a regulator of these early stages of folliculogenesis.
When fetal ovaries were obtained in mid to late gestation (i.e., after day 140), ovarian cortical pieces had mostly primordial follicles. When cortical pieces from mid to late gestation ovaries were cultured with insulin for 2 days, most follicles activated to become primary follicles2‘. Unlike primordial follicles in ovaries before 140 days, they had already acquired the capacity to activate. Similar experiments with ovarian cortical pieces from fetal baboon ovaries yielded similar results23. In the absence of insulin, bovine cortical tissue remained healthy, but activation did not occur in vitro24 and this provided a useful model for studying other potential regulators of follicle activation. We identified kit ligand and bone morphogenetic protein (BMP) 4 as additional factors that promoted activation of bovine primordial follicles and AMH as a hormone that inhibited activation and slowed the growth of primary follicles in vitro20 2’ 2^. It seems likely that whether an individual follicle activates or remains quiescent is determined by the balance of stimulatory vs. inhibitory factors in its microenvironment. A number of such factors have been uncovered in experiments with various species (reviewed in27 28). In the current study, we sought to test the hypothesis that activin A is part of this regulatory system. Learning more about the regulation of primordial follicle activation is important in a practical sense, since the rate of activation affects the size of the ovarian reserve.
The first objective was to test the hypothesis that activin plays a role in follicle formation and in development of the capacity to activate in early to mid-gestation ovaries and also regulates activation in mid to late gestation ovaries, after follicles have formed (Exps. 1 and 3). Since follicle formation and capacity to activate are regulated by ovarian steroids in vitro the second objective was to test the hypothesis that activin modulates steroidogenesis in fetal bovine ovaries (Exp. 2). Objectives 3-5 were focused on examining expression of mRNA for activin A and its type II receptors in fetal ovaries at various developmental stapes and the potential regulation of the mRNAs by estradiol, and on the localization of activin and its type II receptors durinp fetal ovarian development by immunohistochemistry (Exps. 4-6).
Materials and Methods
FETAL OVARIES
Bovine female fetuses at different gestational apes (estimated by crown-rump Ienp th2; gestation = 280 days) were obtained at a local slaughterhouse (Carpill Regional Beef, Wyalusinp, PA). Fetal ovaries were collected and transported to the laboratory in Leibovitz L-15 medium (Life Technologies, Grand Island, NY) supplemented with 1% fetal bovine serum, 50 IU/ml penicillin, and 50 pp/ml streptomycin (Life Technologies) at ambient temperature (20-22 C), as previously described2.
PREPARATION AND CULTURE OF OVARIAN PIECES (EXP. 2) AND OVARIAN CORTICAL PIECES (EXPS. 1, 3, AND 5).
For Exps.1, 3, and 5, ovarian cortical pieces, rich in primordial follicles, were dissected away from the medullary tissue and cut into — 0.5 to 1 mm3 pieces. Four cortical pieces from each fetus were fixed immediately as uncultured controls (day 0). The remaining cortical pieces were placed on uncoated culture well inserts (2 pieces/well; 2 wells/treatment/fetus; Millicell-CM, 0.4-pm pore size; Millipore Corp, Bedford, MA) in the wells of 24-well Costar culture plates (Coming Inc., Corning, NY) with 300 ul Waymouths MB 752/1 medium (Life Technologies) supplemented with 25 mg/L pyruvic acid (Sigma Chemical Co., St. Louis, MO), antibiotics (50 IU/m1 penicillin, 50 Ng/ml streptomycin; Life Technologies), and TS+ (6.25/m1 Ng transferrin, 6.25 ng/ml selenious acid, 1.25 mg/ml BSA, 5.35 pg/m1 linoleic acid; all from Sigma). Ovarian cortical pieces were cultured at 38.5°C in a humidified incubator gassed with 5% CO2: 95% air; 200 pl of culture medium was replaced with fresh medium every other day. The cultures were terminated on day 10 of culture. Because Exp 2 was focused on fetal steroidogenesis, whole fetal ovaries (84-114 days old) were cut into - 0.5 to 1 mrrP pieces, to include all ovarian cell types in that experiment. Ovarian pieces were cultured as described above for cortical pieces except that culture well inserts were not used, the medium contained insulin (ITS+ medium), and the medium (300 pl) was collected and completely replaced every other day and stored frozen for later measurement of hormones.
EXPERIMENTAL DESIGNS (EXPERIMENTS 1-3 AND 5)
In the first experiment, the effects of activin A on follicle formation and activation were examined by culturing cortical pieces isolated from ovaries obtained during early to mid-gestation (90 - 110 days) for 10 days, as described above, in control medium (TS+), with insulin (6.25 pg/ml; Gibco, Grand Island, NY) as a positive control for activation, or with activin A (100 ng/ml; R&D Systems, Minneapolis MN). Concentrations of activin A and insulin were chosen based on previous studies 02*‘ 30 3 . The third experiment was conducted to determine whether the effects of activin A on early follicular development observed in Exp. 1 are developmental-stape specific (i.e., specific to early to mid-gestation, when primordial follicles bepin to form in vivo^). In Exp. 3, cortical pieces were isolated from ovaries obtained durinp late gestation (170-200 days) and cultured for 10 days in control medium (TS+) or with insulin (6.25 pp/ml, as a positive control), activin A (100 np/ml), or activin A + insulin. At the end of culture in Experiments 1 and 3, cortical pieces (day 10) were fixed for histological analysis of follicle formation and activation.
Because steroid hormones regulate follicle formation and activation in fetal bovine ovarian tissue in vitro^ 32 and because activin can modulate steroid production in adult ovarian tissue33 34, we hypothesized that activin A modulates fetal ovarian steroidogenesis. In Experiment 2, ovarian pieces (84-114 days of gestation), containing both cortical and medullary cells, were cultured in ITS+ medium (described above) without or with activin A alone or in combination with FSH, LH, and/or testosterone, hormones shown to regulate fetal steroid production in our previous experiments 2 3^. Ovine FSH (NIADDK oFSH17, lot AFP6446C) and LH (NIADDK oLH26, lot AFP5551 B) were used. Culture medium collected every other day was stored frozen.
Estradiol inhibits follicle formation and activation of newly formed primordial follicles^ 8 24. The aim of Experiment 5 was to test whether estradiol alters the abundance of mRNA for activin A and activin receptors. Cortical pieces were isolated from ovaries obtained during early to mid-gestation (100-
120 days) and cultured for 10 days in TS+ medium without or with estradiol (1 PM). The concentration of estradiol was based on previous studies^. At the end of culture (day 10), pieces were snap-frozen for later extraction of RNA for semi-quantitative RT-PCR analysis.
HISTOLOGICAL MORPHOMETRY (EXPS. I AND 3)
Follicle formation, activation, and development were assessed in cortical pieces by histological morphometry as previously described ^ 22 Briefly, cortical pieces were fixed for 1 h in 2.5% glutaraldehyde, 2.5% formaldehyde in 0.075M cacodylate buffer. The pieces were then embedded in LR white plastic and serially sectioned with an ultra-microtome at 2 pm. Every other set of 10 consecutive sections from each cortical piece was mounted on gelatin-coated slides and stained with toluidine blue. To avoid counting the same follicle twice, only one section in each mounted set of 10 consecutive sections was examined, and only follicles with the terminal vesicle present in the section were counted. Usinp criteria defined previously, follicles were classified as primordial (an oocyte surrounded by a sinple layer of flattened pranulosa cells), primary (an oocyte surrounded by a sinple layer of cuboidal pranulosa cells), or secondary (an oocyte surrounded by more than a sinple layer of cuboidal pranulosa cells). Atresia was rare in fetal ovaries at early to mid-gestation and the percentage of atretic follicles was low ( 2q0%) in ovaries at late gestation and was not affected by treatment in vitro.
RADIOIMMUNOASSAYS (EXP. 2)
In Experiment 2, medium in cultures of ovarian pieces was collected and replaced every 2 days throughout the 10-day culture and stored frozen for later analysis of steroid hormones by radioimmunoassay (RIA). Progesterone, androstenedione, and estradiol were measured in culture medium by RIAs long established in our lab3^ 3’. Duplicate aliquots of 5 to 50 Al of culture medium were assayed, with samples diluted when necessary to ensure measurements were within the range of the standard curve. Culture medium was added to standard curves to control for potential cross-reactivity between the antibody and components of the medium. Sensitivity of assays was 6.25 pg/tube for progesterone and androstenedione and 2.5 pg/tube for estradiol. Inter-assay coefficients of variation (COVs) for progesterone, androstenedione, and estradiol RIAs were 9.3, 8.9, and 9.3%, respectively; intra-assay COVs were 7.3, 6.2, and 8%, respectively. Cross- reactivities of the antibodies were described previously3 . Concentrations of steroids were determined in culture medium colle4ed on every second day of culture (i.e., days 2, 4, 6, 8, and 10).
RT-PCR ANALYSIS (EXPS. 4 AND 5)
Total RNA was isolated from 7 fetal bovine ovaries at different gestational ages (88 to 244 days; Exp. 4) and from cortical pieces after 10 days in culture (Exp. 5) using the RNeasy Kit (Qiagen, Inc., Valencia, CA) according to the manufacturer’s instructions. The amount and purity of RNA were determined spectrophotometrically. Generation of complementary DNA was performed with equal amounts of total RNA in a 20 Al reaction mixture, using random primers and Superscript II Reverse Transcriptase (Life Technologies). Then the rea4ion mixture was diluted with 40 Al water and equal amounts of rea4ion mixture from different samples were used in each PCR reaction.
Primer sequences specific for bovine activin A, activin receptor type IIA and IIB (ACVR2A and ACVR2B), and 18S ribosomal RNA (rRNA) have been described and validated previously3 40. The sequences were as follows: activin A forward: 5-GGC CTG GAG TGT GAC GGC-3; activin A reverse: 5-GCA ACC ACA CTC CTC CAC GAT-3; ACVR2A forward: 5- TTG GGA AAGAGA TAG AAC GAA TCG-3; ACVR2A reverse: 5-GCT TAG GAG TTA CTG GAT TCG ACG-3; ACVR2B forward: 5-GGC CCA GAA GTC ACG TAC GA-3; ACVR2B reverse: 5-GAC AGC CAC GAA GTC GTT CG-3; 18S forward: 5-GCT CGC TCC TCT CCT ACT TG-3; 18S reverse: 5-GAT CGG CCC GAG GTT ATC TA-3.
Equal volumes (15 Al) of the PCR products were separated by electrophoresis through 2% agarose gels containing ethidium bromide. In Exp. 5, band intensities were then analyzed by densitometry using the Kodak ID image analysis software (Kodak, Inc., Rochester, NY). Levels of mRNA for activin A and activin receptors were normalized to the level of 18S rRNA for each sample and expressed as percentages of day 0.
IMMUNOHISTOCHEMICAL LOCALIZATION OF ACTIVIN A AND ACTIVIN RECEPTORS IN BOVINE FETAL OVARIES (EXP. 6)
Fetal bovine ovaries at different gestational apes (n = 6-7; 88 to 244 days of gestation) were fixed in Bouin’s solution. All tissue samples were then dehydrated and embedded in paraffin. Paraffin sections of 7 km were cut, mounted on slides coated with poly-L-lysine (Poly-prep slides; Sigma), and dried overnight at 37”C and then for 15 min at 50°C. After deparaffinization in xylene and rehydration, the sections were incubated with 3% H2O2 for 15 min to block endogenous peroxidases. To prevent nonspecific reactions, sections were incubated with serum blocking solution for 30 min at room temperature. Polyclonal goat anti-human activin A (sc-6308; Santa Cruz Biotechnology, Inc., Santa Cruz, CA), ACVR2A (AF340; Novus Biologicals, Littleton, CO), and ACVR2B (AF339; Novus Biologicals, Littleton, CO) antisera were used for immunohistochemical detection of activin A, ACVR2A, and ACVR2B. Briefly, each section was incubated with antibody to activin A at a dilution of 1:200 at 4’C overnight or with antibody to ACVR2A or ACVR2B at concentrations of 10 kg/ml and 15 kg/ml, respectively, for 2 h at room temperature. The antigen/antibody/enzyme complex was detected and visualized using the SuperPicTureT’ Polymer Detection kit (Life Technologies). Diaminobenzidine (DAB) chromogen substrate, which was added for 4 min, produced brown staining. Sections were then counterstained with hematoxylin for 1 min. Negative controls, which were included in each run, consisted of sections adjacent to the sections analyzed, but with the primary antibody replaced with non-immune serum.
STATISTICAL ANALYSIS
In Experiments 1 and 3, the mean numbers of primordial, primary, secondary, and total follicles per section were calculated for freshly isolated (day 0) and cultured cortical pieces and are presented as means * SEM. Differences amonp the means were evaluated by two-way ANOVA, with treatment and fetus as the two factors. In Experiment 2, concentrations of steroids in culture media were summed over time in culture (cumulative values) and analyzed by three-way ANOVA (activin, hormonal treatment, fetus). In Exps. 1-3, data were lop-transformed before statistical analysis when Hartley’s test indicated heterogeneity of variance (non-transformed values are presented for clarity); statistical significance was assigned at P < 0.05. When a significant P-value was obtained with ANOVA, differences amonp individual means were tested with Duncans multiple ranpe test. Semi-quantitative RT-PCR data (Exp. 5), expressed as fold changes relative to control and presented as means * SEM, were analyzed usinp paired I-tests.
Results
EXPERIMENT I: EFFECTS OF ACTIVIN A ON EARLY FOLLICULAR DEVELOPMENT IN CORTICAL PIECES FROM 90- TO I 10-DAY- OLD FETUSES (FIC. I)
The purpose of this experiment was to examine the effects of activin A on follicle formation and activation. In cattle, follicle formation bepins around Day 90 of pestation^. Freshly isolated cortical pieces (day 0) from 90 to 110-day-old bovine fetuses contained mostly ooponia and oocytes, with only a few primordial follicles (Fip. 1). After 10 days in control medium (TS+ medium), most follicles remained at the primordial stape and there was no increase in the number of primordial, primary, or total (primordial + primary) follicles, compared to the day 0 controls (Fig. 1, AC). In contrast, pieces cultured with insulin or activin A had more primordial, primary, and total follicles than day 0 or day 10 controls (P < 0.05; Fig. 1, AC). With activin A, the numbers of both total and primary follicles increased by 2- and 5-fold, respectively, indicating that it stimulated both follicle formation and activation in vitro.
EXPERIMENT 2: EFFECTS OF ACTIVIN A ON STEROID PRODUCTION IN VITRO BY OVARIAN PIECES FROM 84- TO 114-DAY-OLD FETUSES (FIGS 2 AND 3)
Experiment 1 showed that activin stimulated follicle formation and activation in vitro. In contrast, steroids made by the fetal ovary inhibited these processes in previous studies1635. Since activin can modulate steroid production by mouse preantral follicle? and theca cells from bovine and ovine antral follicles34, Experiment 2 was conducted to test the hypothesis that activin modulates steroid production by fetal bovine ovaries. Ovarian pieces, obtained around the time when follicles begin to form (day 90) and when fetal ovaries are actively producing steroids^, were cultured for 10 days in control medium (ITS+ medium) or with LH and/or FSH, without or with activin A (100 ng/ml). Our previous experiments on the roles of gonadotropins in fetal ovarian steroidogenesis showed that both LH and FSH stimulated progesterone production, whereas LH increased androgen production and FSH stimulated aromatization of androgens to estradiol35 42. In Experiment 2, both gonadotropins increased progesterone accumulation over 10 days of culture, compared to control medium, as expected, and activin increased progesterone by 2- to 4-fold in both the absence and presence of gonadotropins (Fig. 2A; P < 0.05). Androstenedione was increased by LH, as expected, but not by LH + FSH because FSH stimulates the aromatization of androgens increased by LH (Fig. 2B). Activin decreased androstenedione accumulation when LH was present (LH, LH + FSH; P < 0.05), but had no effect in control medium or with FSH. As expected, both gonadotropins increased estradiol production (Fig. 2C, P < 0.05). Interestingly, the combination of activin with either or both gonadotropins decreased estradiol by 50% or more (P < 0.05), but activin had no effect in control cultures (Fig. 2C).
In a separate set of experiments, we determined the effects of activin A on progesterone and estradiol accumulation when exogenous testosterone was included in the medium as a precursor for estradiol synthesis. Testosterone alone increased estradiol more than 10•fold, compared with control cultures, and the combination of testosterone + FSH promoted a further increase (Fig. 3B; P < 0.05). Interestingly, activin had no effect on estradiol accumulation in control cultures or with testosterone + FSH, but it increased estradiol in the presence of testosterone alone (Fig. 3B; P < 0.05). The stimulatory effect of testosterone + activin on estradiol production was similar to that of testosterone + FSH (Fig. 3B; P > 0 05) These results, together with those in Fig. 2, suggest that activin suppresses estradiol production by decreasing the supply of androgen precursor, rather than by inhibiting aromatization of androgen to estradiol. As expected, treatment of fetal ovarian pieces with testosterone decreased progesterone accumulation comparedwith controls, whereas activin alone significantly increased progesterone (Fig. 3A; P < 0.05). Surprisingly, the addition of activin overcame the inhibitory effects of testosterone; the combination of activin with testosterone (and testosterone + FSH) dramatically increased concentrations of progesterone to levels similar to activin alone (Fig. 3A; P < 0.05).
EXPERIMENT 3: EFFECTS OF ACTIVIN A ON EARLY FOLLICULAR DEVELOPMENT IN CORTICAL PIECES FROM 170- TO 200-DAY- OLD FETUSES (FIC. 4)
In Exp. 1, activin A stimulated follicle formation and activation in cortical pieces dissected from fetuses in early to mid-gestation (90 and 110 days), when follicle formation is underway and the capacity of primordial follicles to activate is still developing. Primordial follicles bepin to activate in vivo around mid-gestation §140 days) ^. We previously showed that estradiol inhibits follicle formation and activation in bovine ovaries in mid-pestation^, but not in late pestation20. Therefore, in Exp.3, we investigated the effects of activin A on follicular development in cortical pieces from mid to late gestation (170 to 200 days), a time when many follicles have formed and some have activated, to examine whether the effects of activin A are also stape-specific. On day 0, freshly isolated cortical pieces contained mostly primordial follicles and a few primary follicles (Fip. 4 A,B). After 10 days in culture in control medium (TS+ medium), most follicles remained at the primordial stape and there was no increase in the number of primary follicles (Fip. 4 A,B). Compared with day 10 controls, there were 4- to 5-fold increases in the number of primary follicles and a concomitant decrease in the number of primordial follicles in cortical pieces treated with insulin, activin A, or activin A + insulin (Fip. 4 A,B), suggesting that activin A and insulin both promoted follicle activation. Insulin and activin A + insulin, but not activin A alone, increased the number of secondary follicles, although the numbers of secondary follicles were always very low (Fig. 4C). The combination of activin A + insulin had effects on the numbers of primordial, primary, and secondary follicles that were similar to insulin alone (Fig. 4 A-C). In contrast to the results for cortical pieces from 90 to 110 day-old fetuses (Fig. 1), and as expected for ovaries after day 140 of gestation, the total number of follicles did not increase in response to treatment in vitro (Fig. 4D).
EXPERIMENT 4: EXPRESSION OF MRNA FOR ACTIVIN A AND ACTIVIN RECEPTORS IN FETAL BOVINE OVARIES (FIG. 5)
The expression of mRNA for activin A and activin receptors, ACVR2A and ACVR2B, in fetal ovaries ranpinp from 88 to 196 days of gestation, was determined by semi-quantitative RT-PCR. Messenger RNAs for activin A, ACVR2A, and ACVR2B were detected, but their abundance did not appear to change with fetal age (Fig. 5). ACVR2A mRNA appeared more abundant than mRNA for ACVR2B (Fig. 5).
EXPERIMENT 5: EFFECTS OF ESTRADIOL ON THE ABUNDANCE OF MRNA FOR ACTIVIN A AND ACTIVIN RECEPTORS IN CORTICAL PIECES FROM OVARIES IN EARLY TO MID-GESTATION (FIC. b)
The results of Experiment 1 indicated a stimulatory effect of activin A on bovine follicle formation and activation. Because estradiol inhibited follicle formation and activation in cattle** and suppressed activin expression and signaling in the neonatal mouse ova 43 we examined whether estradiol (1 PM ) alters the abundance of mRNA for activin A and its receptors in cortical pieces from 100- to 120-day-old bovine fetal ovaries cultured for 10 days. Semi-quantitative RT-PCR analyses of cortical pieces after 10 days of culture showed no effect of estradiol on the abundance of mRNAs for activin A, ACVR2A, or ACVR2B (Fig. 6).
EXPERIMENT 6: IMMUNOHISTOCHEMICAL LOCALIZATION OF ACTIVIN A AND ACTIVIN RECEPTORS IN FETAL BOVINE OVARIES (FIGS. 7-9)
Because Exp. 4 showed that fetal bovine ovaries express mRNAs for activin A, ACVR2A, and ACVR2B, we next localized activin A, ACVR2A, and ACVR2B in developing fetal bovine ovaries during the second and third trimesters (day 88-244). Localization of Activin A. Activin A was detected in all fetal ovaries examined from 88 to 244 days of gestation. Activin A was localized by immunohistochemistry to the ooponia, to oocytes in ovigerous cords, and to oocytes in follicles at all developmental stapes (Fip. 7 A,C,D). lmmunostaininp was also detected in granulosa cells of primordial, primary, and secondary follicles (Fig. 7 C,D). The emerging presumptive theca cells around secondary follicles also displayed weak immunoreactivity (Fig. 7D). At the antral stage, staining for activin A was typically present in both granulosa and theca cells (Fig. 7E).
Localization of ACVR2A. ACVR2A protein was detected in bovine ovaries across the range of gestational ages examined and displayed a pattern similar to that of activin A. Oogonia, oocytes in ovigerous cords, and oocytes in follicles at all developmental stages showed immunostaining for ACVR2A (Fig. 8 A-C). Positive staining of granulosa cells was observed throughout all stages of follicular development, including primordial, primary, secondary, and antral follicles (Fig. 8 B-D). Staining was also present in theca cells of secondary and antral follicles (Fig. 8 C,D).
Localization of ACVR2B. nterestingly, the localization of ACVR2B differed from that of activin A and ACVR2A. Although immunostaining for ACVR2B was observed in oogonia, oocytes in ovigerous cords, and oocytes in follicles, staining was weaker after follicles reached the secondary stage (Fig. 9 A,C,D). ACVR2B immunoreactivity was very weak in granulosa cells of primordial and primary follicles and was absent in both granulosa and theca cells of secondary and antral follicles (Fig. 9 C-E), consistent with the lower abundance of its mRNA (Fig. 5).
Discussion
The establishment of the pool of resting primordial follicles and its gradual depletion through activation of primordial follicles are processes critical for female fertility, but their mechanisms are poorly understood, especially in species of practical interest like domestic animals and humans. Cattle are an excellent model for studies of follicular development because of their economic importance and the similarities between follicular development and function in cattle and women 3. The results of the current study provide evidence for activin A as a regulator of early follicular development in cattle. Messenger RNA and protein for activin A and its type II receptors were detected in fetal ovaries at all stages examined and activin A stimulated the formation of follicles and their acquisition of the capacity to activate in vitro. Activin A decreased production of estradiol, an inhibitor of follicle formation and capacity to activate^ 2, by fetal ovarian pieces in vitro by inhibiting the production of androgen precursor (androstenedione). This indicates that activin A may affect follicle formation and capacity to activate in vivo, at least in part, by regulating fetal ovarian steroidogenesis. The presence of activin A and its type II receptors in the bovine fetal ovary, together with the effects of activin A on follicle formation and activation and on ovarian steroidogenesis, argues for a stimulatory role for activin A in early bovine folliculogenesis in vivo.
When pieces of bovine ovarian cortex from early to mid-gestation (90-140 days) were cultured in the presence of insulin, new follicles formed; without insulin the ovarian tissue remained healthy, but follicle numbers did not increase20. In the current study, activin A (100 ng/ml) increased the number of follicles during a 10-day culture about 2-fold, similar to the effect of insulin, the positive control (Fig. 1C). This finding is consistent with the report of Bristol-Gould et al. who found that injecting activin A into neonatal mice increased the number of primordial follicles by 30%, although the “excess” follicles were lost before puberty. Activin A promoted oogonial survival and proliferation in cultured ovarian fragments from human fetal ovaries31. Although there is abundant evidence for steroids as negative regulators of follicle formation in both rodents and cattle, much less is known about positive regulators. Therefore, the finding that activin A, which was also detected in bovine Metal ovaries at this stage, simulated follicle formation is an important step forward.
Primary (activated) follicles were not observed in bovine ovaries in vivo until about day 140 of gestation^. When ovarian cortical pieces from early to mid-gestation (90-140 days) were cultured with insulin, primordial follicles did not activate after 2 days in vitro^, whereas they did when ovarian cortical pieces from mid to late gestation (i.e. after day 140) were cultured with insulin for 2 days2. After a longer, 10-day culture period, newly formed primordial follicles had activated^. These results imply that during culture primordial follicles acquired the capacity to activate in the presence of insulin. In the current study, activin A promoted the activation of newly formed primordial follicles, increasing the number of primary follicles about 5-fold over 10 days in vitro (Fig. 1B), again indicating that it may play a critical role in early bovine folliculogenesis in vivo. The dose of activin was chosen based on published reports 0 30 3*; dose-response studies with fetal bovine material would be useful.
Estrogenic steroids and progesterone inhibited follicle formation and activation in ovaries of newborn rodents in vitro . Likewise, we reported that both estradiol and progesterone inhibited follicle formation and acquisition of the capacity to activate in ovarian cortical pieces from fetal ovaries at early to mid- gestation (90-140 days)^. When bovine follicles are formed, prophase I of meiosis is still in progress and the inhibition of follicle activation by estradiol was correlated with an inhibition of the progression of meiotic prophase I to the resting diplotene phase^. Fetal bovine ovarian pieces synthesize steroids in vitro and their capacity to produce steroids, especially estradiol, declines dramatically around the time that follicle formation begins (day 90 of gestation)^. Steroid synthesis by ovarian pieces was stimulated by LH and FSH in vitro and increasing endogenous steroid production in vitro by adding gonadotropins to the culture medium inhibited follicle formation and activation 2 35, suggesting that steroids are negative regulators of follicle formation and capacity to activate in vivo.
Because activin modulated steroidogenesis in antral follicles33 34, we hypothesized that it promotes follicle formation and capacity to activate in fetal bovine ovaries, at least in part, by modifying the steroid milieu within the fetal ovary. The results showed that activin A had dramatic effects on gonadotropin-stimulated steroidogenesis, decreasing androstenedione and estradiol accumulation in the medium, but increasing progesterone (Fig. 2). The decrease in estradiol appeared to be due to lack of androgen precursor, since activin A did not inhibit estradiol when testosterone was included in the medium as a precursor for estradiol synthesis (Fig. 3B). Our previous studies on the regulation of fetal ovarian steroidogenesis suggested that bovine fetal ovaries use the delta 5 pathway to synthesize androgens, thus bypassing progesterone in the pathway of steroid biosynthesis 35. Use of the delta 5 pathway is consistent with the elevation of progesterone and concurrent inhibition of androstenedione by activin A. The results suggest that activin A inhibits the conversion of progestins to androgens, leading to a decrease in estradiol and thus, to a decrease in its inhibitory effects on follicle formation and capacity to activate.
In contrast to our results (Fig. 4), Cossigny et al.4^ found no effect of activin A on follicle activation in cultured 4-day-old rat ovaries, although the number of preantral follicles increased and atresia of growing follicles decreased. Ding et al.^ reported that activin A (50 ng/ml) decreased activation in human ovarian cortical biopsies cultured for 7 days, but a higher dose (100 ng/ml) had no effect.
Little was known about whether fetal bovine ovaries have activins and their receptors, but the effects of activin A on follicle formation and activation in the current study strongly suggested the presence of ovarian activin receptors. The detection of mRNA for activin A and activin type II receptors (ACVR2A and ACVR2B) in fetal bovine ovaries obtained from late in the first trimester (day 88) to early in the third trimester (day 196) suggest that activin A and its type II receptors are present at least during the second and third trimesters of bovine pregnancy (Fig. 5). Immunohistochemical studies confirmed this hypothesis, revealing the presence of activin A and ACVR2A in germ cells (oogonia and oocytes) and granulosa cells at all stages examined (Figs. 7, 8). Once theca cells were present (secondary and antral follicles), the also stained positively for activin A and ACVR2A (Fig 7 D,E and Fig. 8 C,D). Of the two receptors, ACVR2B and its mRNA were expressed less strongly (Figs. 5, 8, and 9), which suggests that its role in fetal bovine ovaries is secondary to ACVR2A. Although exposure of neonatal mouse ovaries to estrogens suppressed activin expression and signaling 43, culturing bovine ovarian cortical pieces with estradiol in vitro had no significant effect on levels of mRNA for activin A or its type II receptors. Dose-response studies with bovine ovarian tissue might clarify this apparent species difference.
Conclusion
In summary, the results show that activin A stimulated bovine follicle formation and activation in vitro. In addition, activin A decreased the production of estradiol by fetal ovarian tissue, suggesting that regulation of fetal ovarian steroidogenesis may be at least part of the mechanism of activins stimulatory actions. The distinct distribution of activin A and activin receptors in bovine fetal ovaries provides important further evidence for activin A as a regulatory factor in early stages of folliculogenesis in vivo. Future studies on the effects of interactions between stimulators and inhibitors on early bovine follicular development would increase our understanding of the mechanisms of follicle formation and activation, processes critical for female fertility. Because human and bovine ovaries and follicular development are similar, these results may have implications for the establishment and maintenance of the human follicular reserve.
Conflicts of interest:
The authors declare no conflicts of interest.
Funding Statement:
This work was supported by National Institute of Child Health and Human Development Grant HD-060232, Grant no. 2008-35203-05989
from the United States Dept. of Agriculture (USDA) National Institute of Food and Agriculture (NIFA), and by USDA Multistate project NE-1227. Any opinions, findings, conclusions, or recommendations expressed in this publication are those of the author(s) and do not necessarily reflect the view of NIFA or the USDA.
Acknowledgement:
The authors thank Drs G Niswender, T Nett and D T Armstrong for donating the antibodies used for radioimmunoassays, the National Hormone and Pituitary Program NIDDK, NIH, USA for supplying LH and FSH, and Cargill Regional Beef (Wyalusing, PA, USA) for donating the fetal ovaries.
References:
1. Yinp SY. Inhibins, activins, and follistatins: ponadal proteins modulating the secretion of follicle-stimulating hormone. Endocr Rev. May 1988;9(2):267-93. doi:10.1210/edrv-9-2-267
2. Linp N, Ueno N, Yinp SY, et al. Inhibins and activins. Vitam Horm. 1988;44: 1-46. doi:10.1016/s0083-6729(08)60692-5
3. Knipht PG, Satchell L, Glister C. Intra- ovarian roles of activins and inhibins. Mo/ Ce// Endocrinol. Aug 15 2012;359(1-2):53-65. doi:10.1016/j.mce.2011.04.024
4. Ueno N, Nishimatsu S, Murakami K. Activin as a cell differentiation factor. Prog Growth Factor Res. 1990;2(2):113-24. doi:10.1016/0955-2235(90)90027h
5. Bristol-Gould SK, Kreeper PK, Selkirk CG, et al. Postnatal regulation of perm cells by activin: the establishment of the initial follicle pool. Dev Biol. Oct 1 2006;298(1): 132-48. doi:10.1016/j.ydbio.2006.06.025
6. Ding CC, Thong KJ, Krishna A, Teller EE. Activin A inhibits activation of human primordial follicles in vitro. J Assist Reprod Genet. Apr 2010;27(4):141-7.
doi:10.1007/s10815-010-9395-6
7. Mizunuma H, Liu X, Andoh K, et al. Activin from secondary follicles causes small preantral follicles to remain dormant at the resting stage. Endocrinology. Jan 1999;140(1):37-42. doi:10.1210/endo.140.1.6409
8. McLaughlin M, Teller EE. Oocyte development in bovine primordial follicles is promoted by activin and FSH within a two- step serum-free culture system. Reproduction. Jun 2010;139(6):971-8. doi:10.1530/REP-10-0025
9. Yokota H, Yamada K, Liu X, et al. Paradoxical action of activin A on folliculopenesis in immature and adult mice. Endocrinology. Nov 1997;138(11):4572-6. doi:10.1210/endo.138.11.5526
10. Teller EE, McLaughlin M, Dinp C, Thonp KJ. A two-step serum-free culture system supports development of human oocytes from primordial follicles in the presence of activin. Hum Reprod. May 2008;23(5): 1151-8. doi:10.1093/humrep/den070
11. Silva JR, Tharasanit T, Taverne MA, et al. The activin-follistatin system and in vitro early follicle development in poats. J Endocrinol. Apr 2006;189(1):113-25. doi:10.1677/joe.1.06487
12. Ethier JF, Findlay JK. Roles of activin and its signal transduction mechanisms in reproductive tissues. Reproduction. May 2001; 121(5):667-75. doi:10.1530/rep.0.1210667
13. Campbell BK, Souza C, Gong J, et al. Domestic ruminants as models for the elucidation of the mechanisms controlling ovarian follicle development in humans. Reprod Suppl. 2003;61:429-43.
14. Peters H. The development of the mouse ovary from birth to maturity. Acta Endocrinol (Copenh). Sep 1969;62(1):98-116. doi:10.1530/acta.0.0620098
15. Pedersen T. Follicle growth in the immature mouse ovary. Acta Endocrinol (Copenh). Sep 1969;62(1):117-32. doi:10.1530/a4a.0.0620117
16. Yang MY, Fortune JE. The capacity of primordial follicles in fetal bovine ovaries to initiate growth in vitro develops during mid- gestation and is associated with meiotic arrest of oocytes. Biol Reprod. Jun 2008;78(6):1153-61. doi:10.1095/biolreprod.107.066688
17. Russe I. Oopenesis in cattle and sheep. Bibl Anat. 1983;24:77-92.
18. Kezele P, Skinner MK. Regulation of ovarian primordial follicle assembly and development by estropen and progesterone: endocrine model of follicle assembly. Endocrinology. Aug 2003;144(8):3329-37. doi:10.1210/en.2002-0131.
19. Chen Y, Jefferson WN, Newbold RR, Padilla-Banks E, Peplinp ME. Estradiol, progesterone, and penistein inhibit oocyte nest breakdown and primordial follicle assembly in the neonatal mouse ovary in vitro and in vivo. Endocrinology. Aug 2007;148(8):3580-90. doi:10.1210/en.2007-0088
20. Fortune JE, Yanp MY, Muruvi W. In vitro and in vivo regulation of follicular formation and activation in cattle. Reprod Fertil Dev. 2011;23(1):15-22. doi:10.107 1/RD10250
21. Allen JJ, Fortune JE. Formation and activation of follicles in fetal bovine ovarian cortex in vitro are inhibited by increasing endogenous estradiol and progesterone. In: Proceedings from the 47th annual meeting of the Society for the Study of Reproduction; July 19-23, 2014; Grand Rapids, MI. Abstract 388.
22. Wandji SA, Srsen V, Voss AK, Eppig JJ, Fortune JE. Initiation in vitro of growth of bovine primordial follicles. Biol Reprod. Nov 1996; 55(5):942-8. doi:10.1095/biolreprod55.5.942
23. Wandji S, Srsen V, Nathanielsz P, Eppig J, Fortune J. Initiation of growth of baboon primordial follicles in vitro. Human Reproduction. 1997;12:1993-2001.
24. Fortune JE, Yanp MY, Muruvi W. The earliest stapes of follicular development: follicle formation and activation. Soc Reprod Fertil Suppl. 2010;67:203-16. doi:10.73 13/upo9781907284991.018
25. Yanp MY, Cushman RA, Fortune JE. Anti- Mullerian hormone inhibits activation and growth of bovine ovarian follicles in vitro and IS localized to growing follicles. Mo/ Hum Reprod. May 1 2017;23(5):282-291.
doi:10.1093/molehr/gax010
26. Fortune JE, Mouttham LL. Bone morphogenetic protein-4 (BMP-4) promotes activation of bovine primordial follicles in vitro. In: Proceedings from the 46th annual meeting of the Society for the Study of Reproduction; July 22-26, 2013; Montreal, Canada. Abstract 553.
27. Fortune JE. The early stages of follicular development: activation of primordial follicles and growth of preantral follicles. Anim Reprod Sci. Oct 15 2003;78(3-4):135-63. doi:10.1016/s0378-4320(03)00088-5
28. Skinner MK. Regulation of primordial follicle assembly and development. Hum Reprod Update. Sep-Oct 2005;11(5):461-71. doi:10.1093/humupd/dmi020
29. Evans HE, Sack WO. Prenatal development of domestic and laboratory mammals: prowth curves, external features and selected references. Zentralbl Veterinarmed C. Mar 197J;2(1):11-45. doi:10.1111/j.1439-0264.1973.tb00253.x
30. McLaughlin M, Bromfield JJ, Albertini DF, Teller EE. Activin promotes follicular integrity and oopenesis in cultured pre-antral bovine follicles. Mol Hum Reprod. Sep 2010;16(9):644- 53. doi:10.1093/molehr/paq021
31. Martins da Silva SJ, Bayne RA, Cambray N, Hartley PS, McNeilly AS, Anderson RA. Expression of activin subunits and receptors in the developing human ovary: activin A promotes perm cell survival and proliferation before primordial follicle formation. Dev Biol. Feb 15 2004;266(2):334-45. doi:10.1016/j.ydbio.2003.10.030
32. Nilsson EE, Skinner MK. Progesterone regulation of primordial follicle assembly in bovine fetal ovaries. Mol Cell Endocrinol. Dec 10 2009;3 13(1-2):9-16. doi:10.1016/j.mce.2009.09.004
33. Wrathall JH, Knipht PG. Effects of inhibin- related peptides and oestradiol on androstenedione and progesterone secretion by bovine theca cells in vitro. J Endocrinol. Jun 1995;145(3):491-500. doi:10.1677/joe.0.1450491
34. Younp JM, Henderson S, Souza C, Ludlow H, Groome N, McNeilly AS. Activin B is produced early in antral follicular development and suppresses thecal androgen production. Reproduction. May 2012;143(5):637-50. doi:10.1530/REP-11-0327
35. Allen JJ, Herrick SL, Fortune JE. Regulation of steroidogenesis in fetal bovine ovaries: differential effects of LH and FSH. Mol Endocrinol. Nov 2016;57(4):275-286. doi:10.1530/JME-16-0152
36. Berndtson AK, Vincent SE, Fortune JE. Low and high concentrations of gonadotropins differentially regulate hormone production by theca interna and granulosa cells from bovine preovulatory follicles. Biol Reprod. Jun 1995; 52(6):1334-42. doi:10.1095/biolreprod52.6.1334
37. Fortune JE, Hansel W. Modulation of thecal progesterone secretion by estradiol-17 beta. Adv Exp Med Biol. 1979;112:203-8.
38. Fortune JE, Hansel W. Concentrations of steroids and ponadotropins in follicular fluid from normal heifers and heifers primed for superovulation. Biol Reprod. Jun 1985;32(5): 1069-79. doi:10.1095/biolreprod32.5.1069
39. Yoshioka K, Takata M, Taniguchi T, Yamanaka H, Sekikawa K. Differential expression of activin subunits, activin receptors and follistatin penes in bovine oocytes and preimplantation embryos. Reprod Fertil Dev. 1998;10(3):293-8. doi:10.1071/r98011
40. Hirai S, Matsumoto H, Moriya NH, Kawachi H, Yano H. Follistatin rescues the inhibitory effect of activing A on the differentiation of bovine preadipocyte. Domest Anim Endocrinol. Oct 2007;33(3):269-80. doi:10.1016/j.domaniend.2006.06.001
41. Smitz J, Cortvrindt R, Hu Y, Vanderstichele H. Effects of recombinant activin A on in vitro culture of mouse preantral follicles. Mo/ Reprod Dev. Jul 1998;50(3):294-304. doi:10.1002/(SICI)1098-2795(199807)50:3<294::AID-MRD5>3.0.CO;2-E
42. Fortune JE, Yanp MY, Allen JJ, Herrick SL. Triennial Reproduction Symposium: the ovarian follicular reserve in cattle: what regulates its formation and size? Anim Sci. Jul 2013; 91(7):3041-50. doi:10.2527/jas.2013-6233
43. Kipp JL, Kilen SM, Bristol-Gould S, Woodruff TK, Mayo KE. Neonatal exposure to estrogens suppresses activin expression and sipnalinp in the mouse ovary. Endocrinology. May 2007;148(5): 1968-76. doi:10.1210/en.2006-1083
44. Cossipny DA, Findlay JK, Drummond AE. The effects of FSH and activin A on follicle development in vitro. Reproduction. Feb 2012;143(2):221-9. doi:10.1530/REP-11-0105
Author Area
Have an article to submit?
Submission Guidelines
Submit a manuscript
Become a member