Home > Medical Research Archives > Issue 149 > Prenatal Stress and Endo-crine Disrupting Chemical Exposure: Hypothalamic-Pituitary-Adrenal Axis Dysregulation as a Mecha-nism for the Health Conse-quences of Both
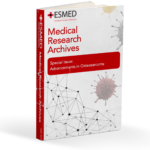
Published in the Medical Research Archives
Jun 2023 Issue
Prenatal Stress and Endo-crine Disrupting Chemical Exposure: Hypothalamic-Pituitary-Adrenal Axis Dysregulation as a Mecha-nism for the Health Conse-quences of Both
Published on Jun 26, 2023
DOI
Abstract
During the past several decades there has been increasing attention to the risks of exposure to endocrine disrupting chemicals, agents that mimic or block the effects of endogenous hormones. Previous research demonstrates that there may be critical periods of development where factors such as prenatal stress and endocrine disrupting chemical exposure can result in endocrine system dysregulation which manifests both immediately and later in life. This review describes the types of common endocrine disrupting chemicals and routes of exposure, the structure and functions of the hypothalamic-pituitary-adrenal axis and its role in the physiological response to stress, and highlights the current evidence showing that endocrine disrupting chemicals may alter normal hypothalamic-pituitary-adrenal axis functions. These topics are unified upon discussion of evidence indicating that prenatal endocrine disrupting chemical exposure has many of the same effects as prenatal stress on the hypothalamic-pituitary-adrenal axis, leading to long-term dysregulation of the axis and subsequent alterations in physiological responses to stress. We further suggest that prenatal endocrine disrupting chemical exposure in combination with prenatal stress may result in additive, if not synergistic effects on the hypothalamic-pituitary-adrenal axis-mediated stress response. Finally, we discuss vulnerable populations at an elevated risk for dual stress and endocrine disrupting chemical exposure and emphasize critical areas for future research.
Author info
1. Introduction to Endocrine Disrupting Chemicals
Endocrine-disrupting chemicals (EDCs) are ex- ogenous chemicals that interfere with hormone ac- tions, and which can be encountered through food and water, consumer products, medications, natural sources, industrial products, pesticides, or occupa- tional exposure 1,2 (Figure 1). The Endocrine Society, in their second Scientific Statement on EDCs, defined an EDC as an exogenous chemical, or mixture of chemicals, that interferes with any aspect of hor- mone action 2. The pool of implicated substances with endocrine disrupting activities continues to ex- pand with advancing research and has an indeter- minate reach. Some of the most well-studied EDCs that are discussed in this review are listed below:
• Bisphenol A (BPA), found in various plastics
• Phthalates, used in plasticizers and personal care products
• Polychlorinated biphenyls (PCBs), in coolants and lubricants of electrical equipment
• Perfluorooctanesulfonic acid (PFOS), found in various consumer products: food packaging, carpeting and upholstery, cleaning, and personal care products
• Organophosphates (OPs), used in pesticides
• Dichlorodiphenyltrichloroethane (DDT), in pesticides
Endocrine disrupting chemicals have been impli- cated in a variety of pathologic processes, and their adverse effects may be most significant when ex- posure occurs during early development when sys- tems are changing most rapidly1,3. The purpose of this review is to examine how prenatal exposure to EDCs can result in life-long effects that are very sim- ilar to those that result from prenatal stress. The im- plications for this are that prenatal exposure to both stress and EDCs might have additive or syner- gistic effects on the developing HPA axis, and to- gether, be a greater risk factor for future disease than either alone. We would like to propose that studies be designed specifically to examine whether both types of prenatal exposures result in effects that are different from either alone.
Figure 1. Known source of Endocrine Disrupting Chemical (EDC) from which humans may be exposed
2. Prevalence of Endocrine Disrupting Chemicals
There are diverse routes of EDC exposure in- cluding ingestion of food, dust, water, and breast milk, skin contact, inhalation of gases and particles, and biological transfer across the placenta. EDCs have been detected in both children and adults in numerous fluids including blood, sweat, urine, breast milk and hair. Pervasive, detectable levels can be found in the blood, serum, and urine of pregnant individuals4,5. Of additional concern, are persistent EDCs, including PCBs, DDT, and PFOS which have been almost universally detected in study samples despite production or use having been banned in the United States for many years5,6. Phthalates are also ubiquitous EDCs; 95–98% of women and chil- dren tested positive for phthalates in two National Health and Nutrition Examination Surveys con- ducted from 1999 to 20147.
3. The Hypothalamic-Pituitary-Adrenal Axis
The hypothalamic-pituitary-adrenal (HPA) axis is a network of endocrine organs which regulate glucocorticoid secretion and facilitate the stress response8. While acute HPA axis activation results in adaptive physiological responses to chal- lenge, chronic or repeated activation can produce adverse effects on many physiological systems9,10. Acute stressors have been characterized as resulting in allostasis, the result of processes which promote adaptation, while chronic stress results in allostatic load, in which homeostatic processes become over- whelmed11. Activation of the HPA axis is initiated upon perception of an external or internal stressor, or threat to homeostasis which causes the release of corticotropin releasing factor (CRF) from the para- ventricular nucleus (PVN) of the hypothalamus to act on corticotrophs of the anterior pituitary12. The pi- tuitary in turn synthesizes and secretes adrenocorti- cotropic hormone (ACTH) 13. ACTH binds the mela- nocortin 2 receptor in the zona fasciculata of the adrenal cortex, stimulating the production and re- lease of glucocorticoids which bind to mineralocor- ticoid (MR) and glucocorticoid receptors (GR) at many sites throughout the body14,15. Negative feed- back to both the levels of the pituitary and the PVN allows for tight regulation of glucocorticoid produc- tion and secretion16,17 (Figure 2).
Figure 2. The hypothalamic-pituitary-adrenal axis. Negative feedback mechanisms are in place at each organ level. Measured HPA axis dysregulation associated with pre-natal stress and EDC exposure have been observed at all levels of regulation.
The HPA axis and its feedback mechanisms are subject to dysregulation, particularly following chronic or repeated stress9,10. Glucocorticoids are essential for the normal development of many or- gan systems and the timing and amount of glucocor- ticoid secretion are critical factors18. Dysregulation of the HPA axis, including that which results from prenatal toxins, can result in adverse health out- comes and a wide spectrum of human disease, from depression to cardiovascular disease to type II dia- betes and metabolic syndrome later in life19-21.
4. Prenatal Stress Effects and the Hypothalamic- Pituitary-Adrenal Axis
The role of prenatal stress on the subse- quent dysregulation of HPA axis function and con- comitant disease has been the subject of a number of excellent reviews22-26. Prenatal stress results in not only acute, but long-term dysregulation of the HPA axis. Glucocorticoids, including those released in response to prenatal stress, can have a long-last- ing effect on many regions of the developing brain27. Maternal glucocorticoids can cross the pla- centa and affect programming of the HPA axis as well as that of brain regions such as the hippocam- pus and amygdala, that modulate the activity of the PVN28. Generally, prenatal stress in rodents results in enhanced HPA responses to an acute stressor later in life29-33, an effect that is often found to be sex-specific23,34-36, though not always37. The nota- ble sex differences are likely due, at least in part, to the interactions between the HPA and hypotha- lamic-pituitary-gonad axes in the developing brain22. Specific effects on the fetus which appear to be maintained into adulthood include higher than normal levels of CRF, altered CRF1 receptor density, enhanced glucocorticoid responsivity and reduced glucocorticoid receptor density23. In rodent studies, a number of often sex-dependent changes in the responsivity or basal activity of components of the HPA axis have been found. Maternal stress results in lower levels of mRNA for POMC in female but not male offspring36. In response to a stressor, adult female rats that had experienced PNS, show ele- vated levels of CRF mRNA in the PVN30, unless the rats were selected for a high anxiety-like behavior phenotype38. Application of a stressor in adult male rats that had undergone prenatal stress causes more CRF-immunoreactivity in the PVN than in non- prenatally stressed controls39. CRF protein levels are also elevated in the PVN of prenatally stressed rats40, although this effect is not always observed41. In one study, levels of CRF protein in the PVN are also elevated following prenatal stress in male, but not female rats42, while in another, it was female rats which displayed elevated levels of CRF expres- sion43. Despite the differences in some of the results, it is clear that the CRF system is affected by prena- tal stress, and most likely the system is primed to be more highly reactive to stressors later in life.
In humans, prenatal stress has been associated with behavioral changes and neuropsychiatric dis- orders, including depression, anxiety, ADHD, con- duct disorders, conduct disorders, and autism spec- trum disorder later in life44. Prenatal stress does ap- pear to alter HPA axis function in humans as well as in animals, but the direction of the effect in humans in more variable25,45.
Excessive levels of prenatal glucocorticoids al- ter GR density in a number of brain regions that modulate HPA axis activity, most notably the hippo- campus, which normally inhibits that activity, and the amygdala, which normally enhances that activ- ity22,25. Prenatal stress-induced re-programming of subsequent HPA axis function results in a reduction in glucocorticoid negative feedback to the hypo- thalamus and pituitary24,46. This is thought to be caused by reduced hippocampal glucocorticoid re- ceptor (GR) density which limits hippocampus-in- duced inhibition of the PVN34,47-51. However, it has also been shown that GR mRNA levels in the hippo- campus of female, but not male rats, is greater fol- lowing prenatal stress31. In mice, this elevated level of the message for the receptor is seen in both sexes52. GR protein levels in the hippocampus are also higher in prenatally stressed female rats than in controls53. A second possibility is that excessive CRF release results in CRF receptor down-regulation with less ultimate glucocorticoid release. The amyg- dala is also affected by prenatal stress in ways that have the capacity to alter HPA axis function. Spe- cifically, CRF mRNA levels, CRF protein levels and CRF1 receptor densities are greater in the amyg- dala of prenatally stressed rodents than in con- trols31,34,43,54,55. Although this CRF is not directly af- fecting ACTH release, it is affecting the modulatory input of the amygdala over the PVN. As previously reviewed, CRF, its two receptors, and its binding protein are affected in different nuclei of the amyg- dala following prenatal stress22.
There are a number of mechanisms by which maternal stress during pregnancy might affect or re-program the responsivity of the HPA axis in off- spring and there is evidence for each. These include maternal glucocorticoid-induced alterations of the fetal HPA axis and epigenetic, intergenerational gene modifications14,24,26,28,34,45,47,56-60. Hippocam- pal inhibition of the HPA axis may also be affected by prenatal stress via the long-established effects of glucocorticoids on the hippocampus24,48,61,62. Ad- ditionally, prenatally stressed mice show higher lev- els of CRF in the CA3 region of the hippocampus63. Importantly, GR and CRF receptors, as well as CRF mRNA are affected in the F2 generation of dams that were stressed while pregnant64. This transgen- erational transmission is important evidence for the guidance of future studies.
5. Prenatal Endocrine Disrupting Chemical Expo- sure and the Hypothalamic-Pituitary-Adrenal Axis: Evidence from Human and Animal Studies
There is increasing evidence that the offspring of pregnant individuals exposed to endocrine-dis- rupting chemicals may also suffer adverse health outcomes. Here, we highlight the possibility that prenatal EDC exposure may result in adverse health outcomes via a similar mechanism to that of prena- tal stress exposure: through HPA axis dysregulation. Like prenatal stress, prenatal EDC exposure can alter HPA axis programming with health consequences later in life4,65. Prenatal exposure to EDCs ap- pears to disrupt the HPA axis on both the levels of the hypothalamus66 and the adrenals67. Addition- ally, EDCs can directly interact with glucocorticoid receptors68,69. Prenatal BPA exposure has been linked to alterations in basal cortisol levels3,4,70-72, cortisol response to a stressor4,70,71, and behavioral response to a stressor73. Prenatal BPA exposure has also been associated with variations in adrenal mass, composition, and histology70. A series of ad- ditional EDCs have also been observed to disrupt various aspects of the HPA axis. Prenatal PCB ex- posure causes alterations in basal and responsive cortisol72,73, and prenatal PFO contact alters CRF1 receptor gene expression in both the hypothalamus and pituitary74. In many of the cited animal and hu- man studies demonstrating physiologic effects of both prenatal stress and EDC exposure, sexually di- morphic results were appreciated4,70,73. Although outside the scope of this review, further considera- tion of the potential evolutionary and clinical signif- icance of sex-specific differences is warranted.
In addition to the noted effects of prenatal EDC exposure on the components of the HPA axis, the chemicals result in behavioral effects which mimic those produced by stress. These include anxiety-like behaviors in animal models75 as well as anxiety and depression in humans76. A number of neurotransmit- ter systems, as well as HPA axis dysregulation have been implicated in the behavioral effects of EDCs76. Critically, the EDCs may alter the way in which these systems respond to stressors later in life. It has been shown that metals, which are endocrine disruptors, can interact with prenatal stress to affect endocrine and behavioral measures77.
Like prenatal stress, there is evidence that EDCs can cause epigenetic modifications which result in intergenerational changes to the function of the HPA axis78-81. Additionally, prenatal exposure to a mix- ture of EDCs alters the methylation status of the CRF1 receptor gene in the hippocampus of adult mice in a manner correlated with hyperactive be- havioral changes82.
6. Intersection of Poverty, Stress, and Endocrine Disrupting Chemical Exposure
As reviewed above, both prenatal stress and prenatal exposure of EDCs have been shown to al- ter the responsivity of the HPA axis to challenge. This dysregulation at critical periods in development has the potential to produce adverse outcomes later in life. Systemic stressors, such as low socio-economic status can contribute to allostatic load, rendering in- dividuals highly susceptible to disease11. Of partic- ular concern is the potential for prenatal stress and EDC exposure to overlap in the same pregnancy and cause an additive effect. This summative effect may perpetuate health inequity in the most vulner- able populations. Exposure to many EDCs occurs via water systems, work-place chemicals, and through bioaccumulation. While both scientific and public knowledge of the potential effects of EDCs is a lim- iting factor, efforts to avoid exposure are difficult without financial and logistical resources. Unfortu- nately, populations which face the greatest risk for EDC exposure are often those that also experience increased prevalence of chronic stressors, both of which are especially critical during pregnancy.
A review of the results of human studies high- lighted the need to evaluate the risks posed by dual exposure to EDCs and prenatal stress6. Indeed, there is accumulating evidence that prenatal pesti- cide exposure in the context of low socio-economic status can result in pediatric cognitive and immune deficiencies. In CHAMACOS, a study of Latino farm- worker families with high occupational pesticide ex- posures, maternal levels of OPs were measured throughout pregnancy83. Standardized IQ measure- ments at age 7 in offspring whose mothers had highest levels of urinary OPs during pregnancy showed a 13.3-point decline in boys facing eco- nomic adversity compared with those from a more privileged status. Likewise, an 8.5-point abatement in girls from economically disadvantaged families was observed compared with a 4.7 decrement in those facing less adversity. The intersection of eco- nomic stressors and pesticide exposure was further observed in the South African VHEMBE study. Among 674 households, maternal exposure to the insecticide DDT resulted in higher rates of childhood illness among those below the designated South Af- rican poverty line and in those who had experienced poor nutrient intake during pregnancy84. Given these observations, and the critical role of the HPA axis in both neurocognitive development and im- mune function, it is highly plausible that dual expo- sures to chronic stress and EDCs intersect in their abilities to cause dysregulation of the HPA axis.
7. Conclusion
It has been well-established that prenatal stress may result in adverse health outcomes, likely via disruption of the maturation of the HPA axis during critical periods of development. There is increasing evidence to suggest prenatal EDC exposure may have very similar effects, which may occur via shared mechanisms. Increased understanding of the effects of EDCs, as well as state and national-level efforts to limit human exposure, will aid intergener- ational health and protect vulnerable populations.
References:
1. La Merrill MA, Vandenberg LN, Smith MT, Goodson W, Browne P, Patisaul HB, Guyton KZ, Kortenkamp A, Cogliano, VJ, Woodruff TJ, Rieswijkl L, Sone H, Korach KS, Gore AC, Zeise L, Zoller RT. Consensus on the key characteristics of endocrine-disrupting chemicals as a basis for hazard identification. Nat Rev Endocrinol. 2020;16:45-57.
2. Gore AC, Chappell VA, Fenton SE, Flaws JA, Nadal A, Prins GS, Toppari J, Zoller RT. EDC-2: The Endocrine Society\'s Second Scientific State- ment on Endocrine-Disrupting Chemicals. Endo- crinol Rev. 2015;36: E1-E150.
3. Gore AC, Heindel JJ, Zoeller RT. Endocrine dis- ruption for endocrinologists (and others). Endo- crinology. 2006;147(6 Suppl): S1-S3.
4. Giesbrecht GF, Ejaredar M, Liu J, Thomas J, Letourneau N, Campbell T, Martin JW, Dewey
D. Prenatal bisphenol a exposure and dysreg- ulation of infant hypothalamic-pituitary-ad- renal axis function: findings from the APrON co- hort study. Environ Health. 2017;16:47.
5. Woodruff TJ, Zota AR, Schwartz JM. Environ- mental chemicals in pregnant women in the United States: NHANES 2003-2004. Environ Health Perspect. 2011;116:878-885.
6. Padula AM, Monk C, Brennan PA, Borders A, Barrett ES, McEvoy CT, Foss S, Desai P, Al- shawabkeh A, Wurth R, Salafia C, Fichorova R, Varshavsky J, Kress A, Woodruff TJ, Morello- Frosch R. Maternal prenatal exposures to envi- ronmental chemicals and psychosocial stressors- implications for research on perinatal outcomes in the ECHO program – implications for re- search on perinatal outcomes. J Perinatol. 2020;40:10-24.
7. DiGangi, J.; Schettler, T.; Cobbing, M.; Rossi, M. Aggregate Exposures to Phthalates in Humans; Health Care Without Harm: Washington, DC, USA, 2002; p. 50.
8. De Kloet, ER., Joels, M., Holsboer, F. Stress and the brain: From adaptation to disease. Nature Rev Neurosci. 2005;6:463-475.
9. Gold, PW., Chrousos GP. Organization of the stress system and its regulation in melancholic and atypical depression: high vs low CRH/NE states. Mol Psychiat. 2002;7:254-275.
10. Dedic, N., Chen, A., Deussing (2018). The CRF family of neuropeptides and their receptors – mediators of the central stress response. Curr Mol Pharmacol. 2018;11:4-31.
11. McEwen BS, Gianaros PJ. Central role of the brain in stress and adaptation: Links to socioec- onomic status, health, and disease. Ann NY Acad Sci. 2010;1186:190-222.
12. Vale W, Spiess J, Rivier C, Rivier J. Characteri- zation of a 41-residue ovine hypothalamic peptide that stimulates secretion of corticotro- pin and beta-endorphin. Science. 1981;213:1394-1397.
13. Rivier C, Vale W. Modulation of stress-induced ACTH release by corticotropin-releasing factor, catecholamines and vasopressin. Nature. 1983;305:325-327.
14. Mbiydzenyuy NE, Hemmings SMJ, Qulu L. Pre- natal maternal stress and offspring aggressive behavior: Intergenerational and transgenera- tional inheritance. Front Behav Neurosci. 2022; 16:977416.
15. Timmermans S, Souffriau J, Libert C. A general introduction to glucocorticoid biology. Front Im- munol. 2019;10:1545.
16. De Kloet ER, Reul JMHM. Feedback action and tonic influence of corticosteroids on brain func- tion: A concept arising from the heterogeneity of brain receptor systems. Psychoneuroendo- crinol. 1987;12:83-105.
17. Smith SM, Vale WW. The role of the hypotha- lamic-pituitary-adrenal axis in neuroendocrine responses to stress. Dialogues Clin Neurosci. 2006;8:383-395.
18. Moisiadis VG, Matthews SG. Glucocorticoids and fetal programming 1: outcomes. Nat Rev Endocrinol. 2014;10:391-402.
19. Zhang C, Xu D, MacKay H, Abizaid A. A plural- ity of molecular targets: The receptor ecosystem for bisphenol-A (BPA). Horm Behav. 2018;101:59-67.
20. Nicolaides NC, Kyratzi E., Lamprokostopoulou A, Chousos GP, Charmandari E. Stress, the stress system and the role of glucocorticoids. Neuro- immunomod. 2015;22:6-19.
21. Kazakou P, Nicolaides NC, Chrousos GP. Basic concepts and hormonal regulators of the stress system. Horm Res Pediatr. 2023;96:8-16.
22. Brunton PJ. Effects of maternal exposure to so- cial stress during pregnancy: consequences for mother and offspring. Reproduc. 2013;146:175-189.
23. Creutzberg KC, Sanson A, Viola TW,Marchis- ella F, Begni V, Grassi-Oliveira R, Riva MA. Long-lasting effects of prenatal stress on HPA axis and inflammation: A systematic review and multilevel meta-analysis in rodent studies. Neu- rosci Biobehav Rev. 2021;127:270-283.
24. Harris A, Seckle J. Glucocorticoids, prenatal stress and the programming of disease. Horm Behav. 2011;59:279-289.
25. Howland MA, Sandman CA, Glynn LM. Devel- opmental origins of the human hypothalamicpituitary-adrenal axis. Expert Rev Endocrinol Metab. 2017;12:321-339.
26. Ruffaner-Hanson C, Noor S, Sun MS, Solomon E, Enriquez Marquez L., Rodriguez DE, Allan AM, Caldwell KK, Bakhireva LN, Milligan ED. The maternal-placental-fetal interface: Adapta- tions of the HPA axis and immune mediators fol- lowing maternal stress and prenatal alcohol. Exper Neurol. 2022;355:114121.
27. Constantinof A, Moisiadis VG, Matthews SG. Programming of stress pathways: A transgener- ational perspective. J Ster Biochem Mol Biol. 2016;160:175-180.
28. Moisiadis VG, Matthews SG. Glucocorticoids and fetal programming part 2: mechanisms. Nat Rev Endocrinol. 2014;10:403-411.
29. Abe H, Hidaka N, Kawagoe C, Odagiri K, Watanabe Y, Ikeda T, Ishizuka Y, Hashiguchi H, Takeda R, Nishimori T, Ishida Y. Prenatal psy- chological stress causes higher emotionality, de- pression-like behavior, and elevated activity in the hypothalamic-pituitary-adrenal axis. Neu- rosci Res. 2007;59:145-151.
30. Bosch O, Much W, Bredewold R, Slattery DA, Neumann ID. Prenatal stress increases HPA axis activity and impairs maternal care in lactating female offspring: Implications for postpartum mood disorder. Psychoneuroendocrinol. 2007;32:267-278.
31. Brunton PJ, Russell JA. Prenatal social stress in the rat programmes neuroendocrine and be- havioral responses to stress in the adult off- spring: Sex-specific effects. J Neuroendocrinol. 2010;22:258-271.
32. Henry C, Kabbaj M, Simon H, Le Moal M, Mac- cari S. Prenatal stress increases the hypotha- lamic-pituitary-adrenal axis response in young and adult rats. J Neuroendocrinol. 1994;6:341- 345.
33. Jafari Z, Mehla J, Kolb BE, Moharjerani MH. Prenatal noise stress impairs HPA axis and cog- nitive performance in mice. Scientific Rep. 2017;7:10560.
34. Mueller BR, Bale TL. Sex-specific programming of offspring emotionality after stress early in pregnancy. J Neurosci. 2008;28:9055-9065.
35. Sanchez MM, McCormack K, Grand AP, Fulks R, Graff A, Maestripieri D. Effects of sex and early maternal abuse on adrenocorticotropin hormone and cortisol responses to the cortico- tropin-releasing hormone challenge during the first 3 years of life in group-living rhesus mon- keys. Dev Psychopathol. 2010; 22:45-53.
36. Weinstock M, Matlina E, Maor GI, Rosen H, McEwen BS. Prenatal stress selectively alters the reactivity of the hypothalamic-pituitary adren-al system in the female rat. Brain Res. 1992; 595:195-200.
37. McLean MA, Simcock G, Elgbeili G, Laplante DP, Kildea S, Hurrion E, Lequertier B, Cobham VE, King S. Disaster-related prenatal maternal stress, and childhood HPA-axis regulation and anxiety: The QF2011 Queensland flood study. Psychoneuroendocrinol. 2020;118:104716.
38. Bosch OJ, Kromer SA, Neumann ID. Prenatal stress: opposite effects on anxiety and hypo- thalamic expression of vasopressin and cortico- tropin-releasing hormone in rats selectively bred for high and low anxiety. Eur J Neurosci. 2006;23:541-551.
39. Piviana SG, Rakitskaya VV, Akulova VK, Ordyan NE. Activity of the hypothalamic-pitui- tary-adrenal system in prenatally stress male rats on the experimental model of post-trau- matic stress disorder. Bull Exper Biol Med. 2016;160:601-604.
40. Fan JM, Chen XQ, Jin H, Du JZ. Gestational hy- poxia alone or combined with restraint sensi- tizes the hypothalamic-pituitary-adrenal axis and induces anxiety-like behavior in adult male rat offspring. Neurosci. 2009;159:1363-1373.
41. Jezova D, Skultetyova I, Makatsori A, Moncek F, Duncko R. Hypothalamic-pituitary-adreno- cortical axis function and hedonic behavior in adult male and female rats prenatally stressed by maternal food restriction. Stress. 2002;5:177-183.
42. Wang X, Meng FS, Liu ZY, Fan JM, Hao K, Chen XQ, Du JZ. Gestational hypoxia induces sex- differential methylation of Crh1 linked to anxi- ety behavior. Mol Neurobiol. 2013;48:544- 555.
43. Zohar I, Weinstock M. Differential effect of prenatal stress on the expression of corticotro- pin-releasing hormone and its receptors in the hypothalamus and amygdala of male and fe- male mice. J Neuroendocrinol. 2011;23:320- 328.
44. Maccari S, Kruger HJ, Morley-Fletcher S, Szyf M, Brunton PJ. The consequences of early-life adversity: Neurobiological, behavioral and ep- igenetic adaptations. J Neuroendocrinol. 2014;26:707-723.
45. Lautarescu A, Craig MC, Glover V. Prenatal stress: Effects on fetal and child brain develop- ment. Int Rev Neurobiol. 2020;150:17-40.
46. Cottrell EC, Seckl JR. Prenatal stress, glucocorti- coids and the programming of adult disease. Front Behav Neurosci. 2009; 3:article 19.
47. Barbazanges A, Piazza PV, Le Moal M, Mac- cari S. Maternal glucocorticoid secretionmediates long-term effects of prenatal stress. J Neurosci. 1996;16:3943-3949.
48. McEwen BS. Stress and hippocampal plasticity. Ann Rev Neurosci. 1999;22:105-122.
49. Shoener JA, Baig R, Page KC. Prenatal expo- sure to dexamethasone alters hippocampal drive on hypothalamic-pituitary adrenal axis activity in adult male rats. Am J Physiol Regul Integr Comp Physiol. 2006;290:R1366- R1373.
50. Szuran T, Pliska V, Pokorny J, Welzl H. Prenatal stress in rats: Effects on plasma corticosterone, hippocampal glucocorticoid receptors, and maze performance. Physiol Behav. 2000;71:353-362.
51. Welberg LAM, Seckl JR, Holmes MC. Prenatal glucocorticoid programming of brain cortico- steroid receptors and corticotrophin-releasing hormone: Possible implications for behavior. Neurosci. 2001;104:71-79.
52. Pascuan CG, Di Rosso ME, Pivoz-Avedikian JE, Wald MR, Zorrilla Zubilete MA, Genaro AM. Alteration of neurotrophin and cytokine expres- sion in lymphocytes as novel markers of spatial memory deficits induced by prenatal stress. Physiol Behav. 2017;173:144-155.
53. Liao L, Yao X, Huang J, Bai S. Prenatal stress up-regulated hippocampal glucocorticoid re- ceptor expression in female adult rat offspring. Int J Morphol. 202;36:400-405.
54. Ward HE, Johnson EA, Salm AK, Birkle DL. Ef- fects of prenatal stress on defensive withdrawal behavior and corticotropin releasing factor sys- tems in rat brain. Physiol Behav. 2000;70:359- 366.
55. Cratty MS, Ward HE, Johnson EA, Azzaro AJ, Birkle DL. Prenatal stress increases corticotro- pin-releasing factor (CRF) content and release from rat amygdala minces. Brain Res. 1995;675:297-302.
56. Bale TL. Epigenetic and transgenerational re- programming of brain development. Nat Rev Neurosci. 2015;16:332-344.
57. Glover V, O’Connor TG, O’Donnell K. Prenatal stress and the programming of the HPA axis. Neurosci Biobehav Rev. 2010;35:17-22.
58. Palma-Gudiel H, Cordova-Palomera A, Eixarch E, Deuschle M, Fananas L. Maternal psycholog- ical stress during pregnancy alters the epige- netic signature of the glucocorticoid receptor gene promotor in their offspring: a meta anal- ysis. Epigenetics. 2015;10:893-902.
59. Hamada H, Matthews SG. Prenatal program- ming of stress responsiveness and behaviours: Progress and perspectives. J Neuroendocrinol. 2018;31:e12674.
60. McGowan PO, Matthews SG. Prenatal stress, glucocorticoids, and developmental program- ming of the stress response. Endocrinol. 2018;159:69-83.
61. Gray JD, Kogan JF, Marrocco J, McEwen BS. Genomic and epigenetic mechanisms of gluco- corticoids in the brain. Nature Rev Endocrinol. 2017;13:661-673.
62. McEwen BS. Physiology and neurobiology of stress and adaptation: central role of the brain. Physiol Rev. 2007;87:873-904.
63. Lv Y, Chen P, Kuang L, Han Z, Solanki B, Zhou W, Tao F, Chen R, Yao Y. Role of corticotropin- releasing hormone in the impact of chronic stress during pregnancy on inducing depression in male offspring mice. Brain Res. 2020;1747:147029.
64. Grundwald NJ, Brunton PJ. Prenatal stress pro- grams neuroendocrine stress responses and af- fective behaviors in second generation rats in a sex-dependent manner. Psychoneuroen- docrinol. 2015;62:204-216.
65. Mathey LIP. Neuroendocrinology of pregnancy: participation of sex hormones. In Marsh, C. (Ed.). (2021). Reproductive Hormones. IntechOpen. doi: 10.5772/intechopen.91499
66. Walley SN, Roepke TA. Perinatal exposure to endocrine disrupting compounds and the control of feeding behavior-An overview. Horm Behav. 2018;101:22-28.
67. Martinez-Arguelles DB, Papadopoulos V. Mechanisms mediating environmental chemical- induced endocrine disruption in the adrenal gland. Front Endocrinol. 2015;6:article 29.
68. MacKay H, Abizaid A. A plurality of molecular targets: The receptor ecosystem for bisphenol- A (BPA). Horm Behav. 2018;101:59-67.
69. Nesan D, Sewell LC, Kurrasch DM. Opening the black box of endocrine disruption of brain de- velopment: Lessons from the characterization of Bisphenol A. Horm Behav. 2018;101:50-58.
70. Panagiotidou E, Zerva S, Mitsiou DJ, Alexis MN, Kitraki E. Perinatal exposure to low-dose bi- sphenol A affects the neuroendocrine stress re- sponse in rats. J Endocrinol. 2014;220(3):207-218.
71. Poimenova A, Markaki E, Rahiotis C, Kitraki E. Corticosterone-regulated actions in the rat brain are affected by perinatal exposure to low dose of bisphenol A. Neurosci. 2010;167(3):741-749.
72. Zimmer KE, Gutleb AC, Lyche JL, Dahl E, Osham IC, Krogenaes A, Skaare JU, Ropstad E. Altered stress-induced cortisol levels in goats exposed to polychlorinated biphenyls (PCB 126 and PCB 153) during fetal and postnatal development. J Toxicol Environ Health A. 2009;72(3-4):164- 172.
73. Reilly MP, Weeks CD, Topper VY, Thompson LM, Crews D, Gore AC. The effects of prenatal PCBs on adult social behavior in rats. Horm Be- hav. 2015;73:47-55.
74. Salgado-Freiria R, Lopez-Doval S, Lafuente A. Perfluorooctane sulfonate (PFOS) can alter the hypothalamic-pituitary-adrenal (HPA) axis ac- tivity by modifying CRF1 and glucocorticoid re- ceptors. Tox Lett. 2018;295:1-9.
75. Harris EP, Allardice HA, Schenk AK, Rissman EF. Effects of maternal or paternal bisphenol A ex- posure on offspring behavior. Horm Behav. 2018;101:68-76.
76. Wiersielis KR, Samuels BA, Roepke TA. Perina- tal exposure to bisphenol A at the intersection of stress, anxiety and depression. Neurotox Ter- atol. 2020; 79:106884.
77. Sobolewski M, Conrad K, Marvin E, Allen JL, Cory-Slechta DA. Endocrine active metals, pre- natal stress and enhanced neurobehavioral dis- ruption. Horm Behav. 2018;101:36-49.
78. Jacobs MN, Marczylo EL, Guerrero-Bosagna C, Ruegg J. Marked for life: Effects of endocrine disrupting chemicals. Ann Rev Environ Resour. 2017;42:105-160.
79. Streifer M, Core AC. Epigenetics, estrogenic en- docrine-disrupting chemicals (EDCs) and the brain. Advan Pharmacol. 2021;92:73-99.
80. Van Cauwenbergh O, Di Serafino A, Tygat J, Soubry A. Transgenerational epigenetic effects from male exposure to endocrine-disrupting compounds: a systematic review on research in mammals. Clin Epigenet. 2020;12:65
81. Anway MD, Skinner MK. Epigenetic transgener- ational actions of endocrine disruptors. Endocri- onol. 2006;Suppl:S430S49.
82. Di Criscio M, Ekholm Lodahl J, Stamatakis A, Kitraki E, Bakoyiannis I, Repouskou A, Bornehag CG, Gennings C, Lupu D, Ruegg J. A human-rel- evant mixture of endocrine disrupting chemicals induces changes in hippocampal DNA methyla- tion correlating with hyperactive behavior in male mice. Chemosphere.
2023;313:137633.
83. Stein LJ, Gunier RB, Harley K, Kogut K, Bradman A, Eskenazi B. Early childhood adversity poten- tiates the adverse association between prena- tal organophosphate pesticide exposure and child IQ: The CHAMACOS cohort. Neurotoxicol. 2016;56:180–7.
84. Huang J, Eskenazi B, Bornman R, Rauch S, Chevrier J. Maternal Peripartum Serum DDT/E and Urinary Pyrethroid Metabolite Concentra- tions and Child Infections at 2 Years in the VHEMBE Birth Cohort. Environ Health Perspect. 2018;126:067006.
Author Area
Have an article to submit?
Submission Guidelines
Submit a manuscript
Become a member