Home > Medical Research Archives > Issue 149 > Selective Modulation of the Cardiac Autonomic Nervous System: A New Therapeutic Strategy for Cardioinhibitory Vasovagal Syncope
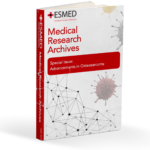
Published in the Medical Research Archives
Jul 2023 Issue
Selective Modulation of the Cardiac Autonomic Nervous System: A New Therapeutic Strategy for Cardioinhibitory Vasovagal Syncope
Published on Jul 06, 2023
DOI
Abstract
Vasovagal syncope (VVS) is the most common type of syncope. The overall prognosis of VVS is usually benign, although the quality of life can be affected when syncope is recurrent and refractory to conventional therapy. Multiple treatment options exist for patients with recurrent VVS, although the effect is modest. In this focused review, we briefly discussed the plausible pathophysiological mechanisms of VVS. We used three case examples to update the current state of cardioneuroablation (CNA) in patients with recurrent cardioinhibitory response as a potential therapeutic option. This article includes review of studies and reports on CNA summarizing the principles of this therapeutic approach; various ways of mapping to localize the parasympathetic ganglionic plexi for ablation; description of technique of extra-cardiac vagal stimulation to confirm parasympathetic denervation of the heart as well as the end points and outcomes of the reported studies. Moreover, we reviewed the pros, cons, gaps, and future directions of this potential therapeutic option in treating patients with VVS.
Author info
Introduction
Vasovagal syncope (VVS) is the most common type of syncope and is more prevalent in women aged 15 to 451. In most cases, VVS is triggered by specific factors, such as prolonged standing, dehydration, or a hot environment, resulting in decreased cardiac preload. However, in some cases it may be caused by emotional triggers. These episodes are usually preceded by a few seconds to minutes of prodromal symptoms such as nausea, sweating, and pallor2. It has been suggested that VVS is mediated by efferent increased parasympathetic activity with subsequent sympathetic withdrawal, causing a varying degrees of bradycardia, reduction in peripheral vascular resistance, and hypotension resulting in cerebral hypoperfusion and syncope. The heart rate and blood pressure patterns recorded spontaneously or induced by tilt table testing define three types of VVS. These are cardioinhibitory response (bradycardia), vaso-depressive response (hypotension), or a mixed type with both cardioinhibitory and vaso-depressive response. A more detailed description of these responses can be found in the Vasovagal Syncope International Study (VASIS) classification3. The mixed response is the most common; the lone cardioinhibitory response is less common. Symptoms are usually more sudden with less prodrome in patients with cardioinhibitory syncope. Injuries and motor vehicle accidents can occur when syncope is abrupt, especially in prolonged bradycardia4. The overall prognosis of VVS is usually benign, although quality of life can be affected when syncope is recurrent with or without associated trauma or injury. There are multiple treatment options for patients with recurrent VVS although the effectiveness is variable.
In this focused review, we will briefly discuss the plausible pathophysiological mechanisms of VVS. Our main goal is to use three case examples to update the current state of cardioneuroablation (CNA) in patients with recurrent cardioinhibitory VVS. We aim to generate a brief discussion of the pros, cons, gaps, and future directions of this potential therapeutic option in treating patients with VVS. The clinical presentation, patient evaluation, and standard management of patients with VVS can be found in a recent consensus statement5 and guidelines6,7 and will not be discussed in this review.
Pathophysiology
The precise mechanism of VVS is still unknown. Most observations suggest that an abnormal or exaggerated autonomic reflex response affecting the heart rate and vasomotor tone can explain the VVS. In a recent review, Garcia et al.8 discussed peripheral and central theory. The peripheral theory involves the heart, blood vessels, baroreceptors, and the Bezold-Jarisch reflex, triggering VVS. This proposed peripheral mechanism suggests that patients with VVS have high parasympathetic activity and diminished sympathetic activity. Baroreceptors located in the inner adventitia of the internal carotid artery and the aorta are deactivated when the blood pools to the lower extremities in the upright position. Baroreceptor deactivation reduces afferent neuronal activity via the glossopharyngeal (carotid sinuses) and vagal nerve (aortic arch) to the cardiovascular regulatory center, the nucleus tractus solitarius in the medulla oblongata of the brain stem. This afferent pathway deactivation releases the vasomotor center inhibiting the vagal and increasing the sympathetic activity, and the peripheral vascular resistance, and cardiac output, preventing syncope. However, in some patients prone to VVS, the sympathetic tone is curtailed; therefore, there is a lack of augmentation of systemic blood pressure. The vigorous myocardial contraction due to reduced preload could activate the ventricular mechanoreceptors. The activation of the mechanoreceptors increases afferent vagal C-fiber neuronal transmission to the cardiovascular regulation center in the brain stem. This afferent activation triggers an efferent sympathetic withdrawal-mediated reduction of peripheral vascular resistance (hypotension), combined with varying degrees of efferent parasympathetic activation-mediated bradycardia, resulting in VVS. These afferent and efferent interactions are known as the Bezold-Jarisch Reflex9,10,11.
The central theory8 proposes that some patients with VVS experience paradoxical cerebral vasoconstriction in response to a reduction in peripheral blood pressure. This paradoxical vasoconstriction further reduces cerebral blood flow resulting in syncope. Emotions can also trigger VVS by activating the limbic system, enhancing sympathetic withdrawal and parasympathetic activation leading to hypotension and bradycardia12. Both peripheral and central theories suggest that the common final pathway for the cardioinhibitory response is mediated by parasympathetic activation.
Cardioneuroablation as A New Therapeutic Strategy for Vasovagal Syncope
The treatment of VVS by cardioneuroablation (CNA) was first discovered by Pachon et al13 which gave origin to the cardioneuroablation that was patented in USA in 2005. It was followed by another study by Pachon et al in 201110 studying long term outcomes of CNA in VVS. From then on, there has been an increasing interest in autonomic modulation for treating VVS in patients with a predominant cardioinhibitory subtype9,11. CNA uses the endocardial radiofrequency ablation technology and technique to ablate the post-ganglionic parasympathetic neurons located in the atrial septum, in the atrial wall, and in the epicardial fat pads. The parasympathetic post-ganglionic neuronal cell bodies are found in the atrial epicardium and in the epicardial fat pads, known as ganglionated plexi (GPs)8. These GPs contain a complex neural network of parasympathetic neuronal cell bodies and sympathetic nerve endings. This complex neuronal network innervates the sinoatrial node (SA node) and atrioventricular node (AV node). These GPs participate and integrate afferent and efferent pathways to and from the cardiac autonomic nervous system, and they also receive inputs from the mechanoreceptors and chemoreceptors8. The highest concentration of GPs is found in the pulmonary veins (Figure 4)14. Some GPs are also located in the ventricle, but these do not play a role in the CNA treatment for VVS. Recent studies have demonstrated that endocardial ablation by targeting the epicardial GPs to modulate the cardiac autonomic regulation of the heart rate could prevent recurrent VVS9,10,14,15.
Methods of Localizing Ganglionic Plexi Several methods have been investigated to locate the GPs. These methods include spectral mapping of AF-Nests, fractionation mapping, anatomically guided approaches, high-frequency stimulation (HFS), and MIBG (123I-metaiodobenzylguanidine) scan. A few key studies are summarized in Table 1. Extra-cardiac vagal stimulation (VS) has been used to control the progression of the denervation, to identify the solid endpoint and to confirm the acute success of CNA. We will provide a brief review of the method used for extra-cardiac VS.
1. Fractionated Electrograms and Spectral Mapping
The Spectral mapping of the AF-Nests and the Fractionated Electrograms were the first methods that gave origin to CNA. Before we discuss the fractionated electrogram (fEGM) and spectral mapping to identify the GPs locations, we would provide a brief description of extra-cardiac vagal stimulation (VS) to assess the endpoint of CNA as the same investigators have used these techniques to identify the ablation sites and to confirm the ablation outcome. Pachon et al.16 first described the VS to assess the outcome of complete parasympathetic denervation. In a prospective study, these investigators conducted VS in 64 patients undergoing radiofrequency ablation for VVS or suspected vagal-mediated atrial fibrillation to achieve parasympathetic denervation. They divided the patients into a denervation group and a control group. The denervation ablation was determined by the complete elimination of the vagal response to the VS. This study found that the VS was reliable and reproducible as it was not changed by anesthesia or by the electrophysiology procedure. No adverse events were reported during a mean follow-up of 8.8 ± 5.0 months. The authors concluded that the VS was feasible, reproducible, and without complications. Patients from the control group who underwent the ablation procedure without parasympathetic denervation had preserved vagal response when the VS was performed at the end of the procedure.
The VS was performed by a radiofrequency ablation catheter positioned in the internal jugular vein (near the jugular foramen) from the distal and the third pole of the quadripolar EP catheter. The stimulator has the following features: direct current (DC) stimulation with square wave pulses of 50 μs (microsecond) in duration, frequency of 30 Hz (Hertz), and amplitude from 10 to 70 V (Volt) (Figure 1). The energy output was adjusted to 0.5 to 1 V/kg (Volt per Kilogram) limited to 70 V, with 30 Hz and a pulse width of 50 μs. The stimulation protocol was performed before and after the ablation procedure. The best response points were marked with fluoroscopy at baseline from either the right or left internal jugular vein. Stimulation from the same location and energy output were repeated post-ablation.
Pachon et al.20 defined two different fractionated EGM (fEGM) types by using spectral analysis: (1) fibrillar atrial myocardium, which may correlate to the epicardial vagal innervation sites, which exhibits a highly fragmented, heterogeneous, and right-skewed frequency distribution; and (2) areas without fragmented signals presumably devoid of epicardial vagal innervation. The investigators called those clusters of fibrillar atrial myocardium AF nests. In normal hearts, these fibrillary EGM may be from neural input present near the venous insertion, interatrial septum, left atrial (LA) roof, or areas overlapping the GPs. In cardiopathy, the fEGM may be also related to fibrosis. This method requires a computer-aided mapping of the entire atrial endocardium. Later in a prospective, controlled, nonrandomized study, Pachon et al.21 enrolled 62 patients in 2 groups: AF nest group (32 patients), including patients with functional or reflex bradyarrhythmias or vagal AF treated with AF nest ablation and a control group (30 patients) with accessory pathways, ventricular premature beats, atrial flutter, atrioventricular nodal reentry, and atrial tachycardia, treated with conventional ablation (non-AFN ablation). In the AF nest group, ablation was delivered at the AF nest determined by the fractionation of EGM and by the 3-dimensional anatomic location of the GPs. The vagal response was evaluated before, during, and post-ablation by extra-cardiac VS. The AF nest map was obtained during anatomy acquisition with the Ensite Velocity system (Abbot, Abbott Park, IL, USA) using the Fractionation Software with Fast Fourier transformation. At baseline, VS induced sinus pauses, asystole, and transient atrioventricular block in both groups (P=0.96). In the AF nest group where the AF-nests were mapped and ablated, VS completely abolished the cardiac vagal response in all cases (pre/post ablation VS =P<0.0001). In the control group, vagal response remained unchanged post-ablation (P=0.35), confirming that non-AF nest ablation did not affect cardiac vagal innervation by evidence of persistence of vagal response to VS. Their results also suggest that AF-nests identified by fractionated EGM and spectral analysis are anatomically related to vagal innervation.
The study by Chen et al.19 investigated patients with symptomatic sinus node dysfunction undergoing CNA guided by fractionated EGM along with VS in real-time before and after the ablation of each GPs. They ablated the GPs located between the aortic root and the medial wall of the superior vena cava (Ao-SVC GP), between the posterior wall of the coronary sinus ostium and the left atrium (PMLGP), between the anterior antrum of the right superior pulmonary vein and the superior vena cava (RAGP) and in the superolateral area around the root of the left superior pulmonary vein (LSGP). The investigators showed that the median heart rate increased from 52.8 ± 2.1 bpm at baseline to 73.0 ± 10.4 bpm after the procedure (p = 0.012) and to 71.3 ± 10.1 bpm at the six-month follow-up (p = 0.011) evaluated by Holter monitoring. Bradycardia-related symptoms subsided in all patients at the six-month follow-up.
2. Anatomically Guided Cardioneural Ablation
As the name suggests, this technique leverages our knowledge of the typical locations of the cardiac GPs to perform CNA. In 2016, Sun et al.14 enrolled 57 consecutive patients (aged 43.2±13.4 years; 35 women) with refractory VVS. High-frequency stimulation (HFS) and anatomically guided GP ablation were performed in 10 and 47 cases, respectively. A positive vagal response was defined when HFS induced any of the following phenomena: transient ventricular asystole, atrioventricular block, or an increase in mean R‐R interval of 50%. The endpoint of the HFS-guided ablation was the elimination of all vagal responses at each identified target. The anatomically guided ablation protocol included ablation of the left superior GPs (located in the left atrial roof outside the left superior pulmonary vein), left inferior GPs (inferior and posterior to the ostium of the left inferior pulmonary vein), right anterior GPs (anterior and superior to the right superior pulmonary vein), right inferior GPs (inferior and posterior to the ostium of the right inferior pulmonary vein) and the left lateral GPs (located between the left atrial appendage and the left inferior pulmonary vein). At each anatomically guided GPs site, if a test radiofrequency energy delivery induced any vagal response (defined as transient ventricular asystole, atrioventricular block, or an increase in mean R‐R interval of 50%) within 10 seconds, further energy was delivered for at least 30 seconds until inhibition of the vagal response. Otherwise, the testing ablation was considered to be completed at that site. Additional ablation was then performed adjacent to the initial lesion to form a cloudlike lesion cluster. The endpoint of the anatomically guided procedure was defined as follows: once at each GP site, five consecutive ablation attempts failed to induce any vagal response. During follow-up of 36.4±22.2 months (range 12-102 months), 52 patients (91.2%) remained free from syncope. No statistical differences were found between the HFS- and anatomically guided ablation groups in freedom from syncope or recurrent prodromes.
In 2019, Hu et al.17 enrolled 115 patients with VVS who underwent CNA. Left atrial GPs were identified by HFS and/or anatomic landmarks for radiofrequency catheter ablation. They observed no syncope recurrence in 92.2% of the patients during a follow-up of 18 months. The authors concluded that CNA, guided by HFS or anatomy, in the LA effectively reduced the recurrence of VVS. They also observed that ablation of the right anterior plexus (located between the aortocaval fad pad and the right anterior GPs) resulted in increased sinus rates immediately after the procedure and during follow-up.
3. High-Frequency Stimulation
Prior to the non-invasive MIBG scan, the localization of GPs has been demonstrated by invasive multi-site testing with high-frequency stimulation (HFS)23,24,25, a method that identifies GPs by their typical vagal (parasympathetic) response of bradycardia by sinus rate or slowing atrioventricular nodal conduction. The method of HFS-based CNA was first studied by Yao et al.9. The authors reported that the application of HFS causes two different responses in the atria: (1) a vagal response diagnosed with a significant prolongation of the PR or RR intervals; (2) a lack of response characterized by the absence of any effect or non-significant changes on the PR or RR intervals. These responses demonstrate vagal or non-vagal innervation sites, respectively. The stimulation delivers a frequency of 20 Hz, amplitude of 0.1-1 mA or 10-30 V, and pulse duration of 1-10 ms for 5 seconds at each site. The investigators performed ablation guided by this method on ten consecutive patients (mean age, 50.4±6.4 years; 7 women) with a mean of 3.5 (range, 2–20) episodes of VVS during the preceding year and positive head-up tilt testing in whom standard therapies were ineffective or poorly tolerated. GPs in the LA, identified by high-frequency stimulation, were targeted by radiofrequency catheter ablation. The endpoint of the procedure was an elimination of the vagal response at each target after at least 60 seconds of radiofrequency energy delivery. At 30±16 (range, 13–55) months of follow-up, no patient had any recurrence of syncope, and all patients had significant improvement in symptoms, but 5 of 10 patients reported transient prodromes of lightheadedness. No complications occurred. However, investigators reported that HFS might cause discomfort, especially with increasing voltage, due to a specific excitation of nociceptors within the wall of the atria, SVC, or CS. Most conscious patients could not tolerate stimulation of more than 15 V. Applications with high amplitude may cause inadvertent AF induction requiring additional cardioversions and atrial stunning.
4. Metaiodobenzylguanidine scan
Stirrup et al.18 reported that 123I-metaiodobenzylguanidine (123I-mIBG) solid-state SPECT LA innervation imaging (LAII) has the spatial resolution to detect left atrial GPs non-invasively. It can accurately and reproducibly identify GPs verified by HFS, particularly when reconstructed with cardiorespiratory gating. They included 20 patients in the study who underwent atrial fibrillation (AF) ablation. Apart from its use in atrial fibrillation, this method has not been widely used for CNA other than in a case report by Romanov et al.22 The authors showed that using image acquisition after injection of I-MIBG provides a 3D cardiac electro-anatomical map that could identify target sites for GP ablation.
Case Review
We present three patients with recurrent VVS and documented pauses who were managed at Hospital do Coração, São Paulo, Brazil.
Case 1
An 18-year-old male presented with frequent and recurrent syncope. All episodes were preceded by prodromal symptoms such as nausea, fatigue, and warmth, consistent with VVS. Conventional treatment, such as liberalization of salt and water intake, compression stockings, and avoiding triggers or medications, did not alleviate syncope. Holter monitoring and tilt table test showed sinus arrest/sinus pause suggesting cardioinhibitory VVS (Figure 2). Due to recurrent symptoms affecting the quality of life and failing conventional medical therapies, a shared decision with the patient was made prior to undergoing CNA.
He was taken to the electrophysiology laboratory in a fasting state and placed under general anesthesia. Extra-cardiac VS was performed at baseline, showing sinus arrest/sinus pause (Figure 3, Panel 3A). The ablation was performed anatomically at the target location of GPs which consists of aortocaval junction (GP 1), anterior right superior pulmonary vein (RSPV), and adjacent right atrium at the Waterston’s sulcus (GP2), between right inferior pulmonary vein, mitral valve and adjacent inferoposterior right atrium (GP 3) and near the left superior pulmonary vein roof (GP4) (Figure 4). At the end of ablation, the extra-cardiac VS showed no inducible sinus arrest/sinus pause meeting the pre-procedural defined endpoint (Figure 3, Panel 3B). A heart rate (sinus rate) increase was noted post-ablation after the vagal denervation (Figures 3, Panel 3C and 3D). The patient was followed for 24 months without recurrence of syncope. Holter monitoring on follow-up showed no sinus arrest/sinus pauses (Figure 5). There were no acute or long-term complications noted.
Case 2
A 65-year-old man with a history of hyperlipidemia and carotid atheroma presented with recurrent syncope episodes preceded by nausea and visual changes. His vital signs were stable, and his cardiopulmonary exam was unremarkable. Laboratory tests and electrocardiogram were normal; an echocardiogram showed a grade I diastolic dysfunction. A 24-hour Holter monitoring showed no pauses or arrhythmia. The average heart rate was 61 beats per minute, ranging from 40 to 117 bpm. A tilt table test showed a mixed response associated with syncope (Figure 6, Panel 6A). Carotid sinus massage induced a 5.8-second sinus pause (Figure 7). CNA was performed with a similar technique of extracardiac VS as in Case 1. In this case, ablation was performed at the anterior right GP (GP2) and the aortocaval ganglia (GP1), after which the vagal response was abolished; hence further ablation was not required. The patient had no recurrent syncope during a follow-up of 24 months. A repeat tilt table test was negative for cardioinhibitory or vasodepressor response (Figure 6, Panel 6B). Carotid sinus massage was also negative. A 24-hour Holter monitoring showed a heart rate range from 53 to 95 bpm, with an average heart rate of 69 bpm.
Case 3
A 16-year-old male with a history of generalized tonic-clonic seizure and loss of consciousness since the age of 5. He has been treated with anti-epileptic medications. He also had episodes of syncope associated with blood draws or emotional stress. He underwent tilt table testing. A 28-second sinus arrest was induced and correlated with a generalized tonic-clonic seizure (Figure 8, Panel 8A). He underwent CNA with similar techniques as in Case 2 at GP1 and GP2. He has been free of syncope and seizure for over two years without taking any medications (Figure 8, Panel 8B). This case highlighted the importance of recognizing the reported tonic-clonic seizure could be secondary to high vagal tone-mediated sinus arrest and prolonged cerebral hypoperfusion.
Current State of Clinical Evidence
Pachon et al.13 described the first CNA in a total of 5 patients by using spectral mapping to locate the areas with high concentrations of epicardial GPs where fractionated EGMs were observed. They described the ablation of three main GPs: 1. area between the aorta and superior vena cava (SVC) anterior to the right pulmonary vein ( ablated from the superior vena cava); 2. area near the SA node adjacent to the right pulmonary vein atrial junction ( ablated from the left atrium); and 3. area near the AV node, adjacent to the junction of the inferior vena cava (IVC) and the left and right atrium (ablated from the inferior vena cava and the coronary sinus) and also the surface of the left interatrial septum between right PV insertions and the fossa ovalis and also, the surface of the left interatrial septum between right PV insertions and the fossa ovalis. The endpoints of the procedure included the elimination of the potentials with a right spectral shift in the right and left atrial regions surrounding the sinus and AV node, a persistent increase in the sinus rate, and a decrease in the cycle length when the Wenckebach second-degree AV block occurred. The authors13 reported these patients were free from syncope or dizziness except for one patient who later had a positive tilt test. These investigators10 subsequently evaluated a larger cohort of 43 patients with recurrent VVS and significant cardioinhibitory (pauses 13.5 +/- 13 s) response during the tilt test. The study showed reduced recurrent syncope during a follow-up of 45 months. Other cohort studies of CNAs in treating patients with VVS9,11,15 also demonstrated no syncope recurrence during follow-ups between 10 and 30 months. Hu et al.,17 studying a cohort of 115 patients, targeted primarily the right anterior plexus. They observed a significant increase in HR compared to ablating GPs around the pulmonary veins. These investigators reported no syncope recurrence in 92.2% of the patients during a follow-up of 18 months.
A recent systematic review by Vadenberk et al.23 showed that 465 patients with VVS and a mean age of 39.8 +/- 6 4.0 years (53.5% female) underwent CNA. This review included one randomized trial, one non-randomized trial, and twelve observational studies (5 retrospective and 7 prospective). The authors highlighted the large variability in patient selection, ablation methods, definitions of successful procedural outcomes, short- and long-term clinical endpoints. In 13 studies, a tilt table test was performed before the CNA procedure. In 9 studies, the VASIS classification could be extracted: 156 patients (66.1%) had a cardioinhibitory response, 74 (31.4%) a mixed response, and 6 (2.5%) a vasodepressor response. Only 8 studies reported medication used or attempted before CNA; 5 studies included information on medications after CNA. Of the total 465 patients, 50 (10.8%) underwent CNA guided by fractionated electrogram (fEGM), 73 (15.7%) by the spectral method, 210 (45.2%) by HFS, and 73 (15.7%) by an anatomically guided approach. In the remaining patients, 59 (12.6%), a combination of techniques was used, or the exact method could not be determined. Biatrial ablation was performed in 168 patients (36.1%); the remaining were treated by ablation in the left atrium 259 (55.7%) or right atrium only 38 (8.2%). The mean follow-up was 24.6 +/- 11.3 months.
The freedom from syncope at the end of the follow-up was 92.0% (95% CI 88.1%–94.6%). The freedom from prodromes (8 studies; n =301) was 86.0%. There was no difference in subgroup analysis comparing different methods to identify targeted GPs. There was lower freedom from syncope in procedures limited to the right atrium. Overall, procedure-related adverse events were 13%, with AF during HFS and inappropriate sinus tachycardia during follow-up being the most common.
Conclusion and Future Directions
Vasovagal syncope is a common cause of syncope associated with a benign prognosis in most patients. The clinical presentation can be abrupt with minimum prodrome in a subgroup of patients with a cardioinhibitory response. Injuries can be associated with abrupt onset of syncope, and quality of life can be affected in these patients with recurrent episodes not responding to medical therapy. Pacemaker therapy can be helpful in selected patients with cardioinhibitory syncope. However, syncope recurrences and pacemaker-related complications reflect short-term and long-term challenges in younger patients. We had described three cases examples of successful CNA in patients with recurrent cardioinhibitory component along with current state of clinical evidence by summarizing the end points and outcomes of several studies on the CNA using various methods of mapping the GPs and assessing the vagal denervation. CNA is a new therapeutic option for cardioinhibitory syncope, especially for young subjects seeking a one-time and effective treatment. If confirmed to be effective and safe, CNA therapy has significant advantages. Successful ablation of the GPs could have a long-lasting effect on cardiac parasympathetic innervation because the post-ganglionic neuronal cell bodies are affected. Effects on the sympathetic and sensory systems could be transient or short-term, as the damaged post-ganglionic nerve fibers could be repaired by axonal regeneration in a few months24,25,26. These plausible explanations of CNA for VVS require further confirmation of the precise localization of targeted GPs, reproducible procedural endpoints, standardization of ablation targets, and long-term outcomes. Long-term consequences of a plausible permanent parasympathetic denervation are unknown.
Despite the challenges in treating recurrent VVS, most do well without intervention, even with documented prolonged asystole27.VVS episodes tend to occur in clusters in discrete time windows interspersed with long asymptomatic periods28. Most patients seek medical evaluation when their symptoms are in a bad cluster 29. These natural history data suggest that most patients will get better with time. A more conservative approach is reasonable for most patients. Some patients may be prone to the placebo effect30, thus highlighting the importance of sham-controlled trials in this field. Sharma et al.31 reported that an expert intervention (an expert touch) to conventional non-pharmacologic therapy for VVS might accelerate improvement in VVS. Under these contexts, additional data on patient selection, standardization of ablation targets and techniques, and short-term and long-term outcomes will be needed before the adaptation of CNA as a therapeutic option for patients with VVS. These data must be derived from well-designed clinical trials with sufficient sample size and follow-up.
Effectiveness, complications, and side effects need to be compared between CNA and standard therapy. What are the short-term and long-term consequences of a presumed permanent cardio-vagal denervation? Are complications from the CNA compared favorably to side effects from long-term use of medical therapy for VVS? Can the mapping of CNA targets be standardized? What will be the ablation targets, RA, LA, or bi-atrial? As we presented three case examples to highlight the potential usefulness of CNA in patients with cardioinhibitory VVS, further investigation of the questions raised in the review is strongly warranted.
Conflicts of Interest Statement:
The authors have no conflicts of interest to declare.
Funding Statement:
No funding was utilized for the preparation of this article.
References:
1. Marquez NoM.F., et al., Role of the sympathetic nervous system in vasovagal syncope and rationale for beta-blockers and norepinephrine transporter inhibitors. Medwave, 2016. 16(Suppl4): p. e6824.
2. Alboni, P., The different clinical presentations of vasovagal syncope. Heart, 2015. 101(9): p. 674-8.
3. Brignole, M., et al., New classification of haemodynamics of vasovagal syncope: beyond the VASIS classification. Analysis of the pre-syncopal phase of the tilt test without and with nitroglycerin challenge. Vasovagal Syncope International Study. Europace, 2000. 2(1): p. 66-76.
4. Maloney, J.D., et al., Malignant vasovagal syncope: prolonged asystole provoked by head-up tilt. Case report and review of diagnosis, pathophysiology, and therapy. Cleve Clin J Med, 1988. 55(6): p. 542-8.
5. Sheldon, R.S., et al., 2015 heart rhythm society expert consensus statement on the diagnosis and treatment of postural tachycardia syndrome, inappropriate sinus tachycardia, and vasovagal syncope. Heart Rhythm, 2015. 12(6): p. e41-63.
6. Shen, W.K., et al., 2017 ACC/AHA/HRS guideline for the evaluation and management of patients with syncope: A report of the American College of Cardiology/American Heart Association Task Force on Clinical Practice Guidelines and the Heart Rhythm Society. Heart Rhythm, 2017. 14(8): p. e155-e217.
7. Brignole, M., et al., [2018 ESC Guidelines for the diagnosis and management of syncope]. Kardiol Pol, 2018. 76(8): p. 1119-1198.
8. Garcia, A., et al., Cardioinhibitory syncope: from pathophysiology to treatment-should we think on cardioneuroablation? J Interv Card Electrophysiol, 2020. 59(2): p. 441-461.
9. Yao, Y., et al., Endocardial autonomic denervation of the left atrium to treat vasovagal syncope: an early experience in humans. Circ Arrhythm Electrophysiol, 2012. 5(2): p. 279-86.
10. Pachon, J.C., et al., Catheter ablation of severe neurally meditated reflex (neurocardiogenic or vasovagal) syncope: cardioneuroablation long-term results. Europace, 2011. 13(9): p. 1231-42.
11. Debruyne, P. and W. Wijns, Cardio-Neuromodulation: The Right-Sided Approach. JACC Clin Electrophysiol, 2017. 3(9): p. 1056-1057.
12. Reid, I.A., Interactions between ANG II, sympathetic nervous system, and baroreceptor reflexes in regulation of blood pressure. Am J Physiol, 1992. 262(6 Pt 1): p. E763-78.
13. Pachon, J.C., et al.,Cardioneuroablation--new treatment for neurocardiogenic syncope, functional AV block and sinus dysfunction using catheter RF-ablation. Europace, 2005. 7(1): p. 1-13.
14. Sun, W., et al., Catheter Ablation as a Treatment for Vasovagal Syncope: Long-Term Outcome of Endocardial Autonomic Modification of the Left Atrium. J Am Heart Assoc, 2016. 5(7).
15. Aksu, T., et al., Simplified Cardioneuroablation in the Treatment of Reflex Syncope, Functional AV Block, and Sinus Node Dysfunction. Pacing Clin Electrophysiol, 2016. 39(1): p. 42-53.
16. Pachon, M.J., et al., Simplified Method for Vagal Effect Evaluation in Cardiac Ablation and Electrophysiological Procedures. JACC Clin Electrophysiol, 2015. 1(5): p. 451-460.
17. Hu, F., et al., Right anterior ganglionated plexus: The primary target of cardioneuroablation? Heart Rhythm, 2019. 16(10): p. 1545-1551.
18. Stirrup, J., et al., Hybrid solid-state SPECT/CT left atrial innervation imaging for identification of left atrial ganglionated plexi: Technique and validation in patients with atrial fibrillation. J Nucl Cardiol, 2020. 27(6): p. 1939-1950.
19. Chen, W., et al., Extracardiac Vagal Stimulation-Assisted Cardioneuroablation: Dynamically Evaluating the Impact of Sequential Ganglionated Plexus Ablation on Vagal Control of SAN and AVN in Patients with Sinoatrial Node Dysfunction. J Cardiovasc Dev Dis, 2022. 9(6).
20. Pachon, M.J., et al., A new treatment for atrial fibrillation based on spectral analysis to guide the catheter RF-ablation. Europace, 2004. 6(6): p. 590-601.
21. Pachon, M.E., et al., Relation of Fractionated Atrial Potentials With the Vagal Innervation Evaluated by Extracardiac Vagal Stimulation During Cardioneuroablation. Circ Arrhythm Electrophysiol, 2020. 13(4): p. e007900.
22. Romanov, A., et al., Visualization and ablation of the autonomic nervous system Approach. JACC Clin Electrophysiol, 2017. 3(9): p. 1056-1057.
23. Vandenberk, B., et al., Cardioneuroablation for vasovagal syncope: A systematic review and meta-analysis. Heart Rhythm, 2022.
24. Aksu, T., et al., Catheter Ablation of Bradyarrhythmia: From the Beginning to the Future. Am J Med Sci, 2018. 355(3): p. 252-265.
25. Kim, D.T., et al., Sympathetic nerve sprouting after orthotopic heart transplantation. J Heart Lung Transplant, 2004. 23(12): p. 1349-58.
26. Gallego-Page, J.C., et al., Re-innervation after heart transplantation: a multidisciplinary study. J Heart Lung Transplant, 2004. 23(6): p. 674-82.
27. Carvalho, M.S., et al., Prognostic Value of a Very Prolonged Asystole during Head-Up Tilt Test. Pacing Clin Electrophysiol, 2015. 38(8): p. 973-9.
28. Sahota, I.S., et al., Clusters, Gaps, and Randomness: Vasovagal Syncope Recurrence Patterns. JACC Clin Electrophysiol, 2017. 3(9): p. 1046-1053.
29. Sheldon, R.S., et al., Worsening of symptoms before presentation with vasovagal syncope. J Cardiovasc Electrophysiol, 2007. 18(9): p. 954-9.
30. Sahota, I., R. Sheldon, and P. Pournazari, Clinical improvement of vasovagal syncope in the absence of specific therapies: The Seinfeld effect. Cardiol J, 2014. 21(6): p. 637-42.
31. Sharma, G., et al., Effect of Yoga on Clinical Outcomes and Quality of Life in Patients With Vasovagal Syncope (LIVE-Yoga). JACC Clin Electrophysiol, 2022. 8(2): p. 141-149.
Author Area
Have an article to submit?
Submission Guidelines
Submit a manuscript
Become a member