Home > Medical Research Archives > Issue 149 > Gene Therapy Drug Development for First-In-Human Study of Pediatric Diseases: Facilitating Change to Current Paradigms
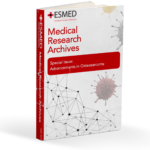
Published in the Medical Research Archives
Jul 2023 Issue
Gene Therapy Drug Development for First-In-Human Study of Pediatric Diseases: Facilitating Change to Current Paradigms
Published on Jul 29, 2023
DOI
Abstract
Drug development in pediatrics is mandated under US and European Union legislation, and delays in pediatric studies can impact the appropriate labeling and use of therapeutics for children. Developing medical products for pediatrics is challenging, as there are several critical issues and factors to consider when initiating a pediatric drug development program. The International Conference Harmonisation guideline E11(R1) and criteria under 21 CFR part 50 subpart D define pediatric regulatory standards for drug developers and ensure the safety of pediatric participants in clinical studies.
Adequate adult data are typically required before finalizing pediatric study designs and initiating pediatric studies by virtue of the Pediatric Research Equity Act. It is evolving that the Food and Drug Administration (FDA) is including adolescents in Phase 3 trials. In pediatrics, the lack of coordinated use of extrapolation for safety and efficacy amongst global regulatory agencies impacts the timelines and development of clinical trial designs. First-in-human pediatric trials may be justified if the aspects of 21 CFR 50, subpart D specifically 21 CFR 50.52 are addressed. For example, first-in-human pediatric gene therapy trials have been allowed in spinal muscular atrophy (SMA) using a benefit-risk assessment to justify the conduct of first-in-human trials in children. The statutory requirement to study children in clinical trials is influenced by the nature of the disease that is currently under study and needs to be personalized. These issues are addressed in this perspective on gene therapy treatment in children.
Author info
Developmental Paradigms Using Extrapolation in Pediatric Drug Development
Clinical drug development in pediatrics is mandated under US and European Union legislation, and delays in pediatric studies can impact health care delivery. Before pediatric studies are completed, adolescents are typically treated with unlicensed, adult-approved therapies, and younger children are prescribed doses that are not adequately supported by data. Post- market studies in pediatrics are often not initiated until several years after adult indication approval, leading to years of unlicensed use without proper safety surveillance. Critical aspects such as pediatric specific endpoints, unique developmental safety concerns and even specific pediatric formulations or routes of administration need to be taken into consideration.
Several factors may contribute to the failure of reaching timely completion of clinical studies in a pediatric population, including the relative rarity of a disease in children, potential requirement for washout periods of other medications, and burden on trial participants, particularly when drugs are accessible off-label and clinical study sites are not readily accessible. Although it is important to collect knowledge on the effects of medicinal products in pediatric patients, this should be done without compromising the safety of pediatric patients participating in clinical studies. It is imperative that special measures are set in place to protect children from inappropriate risk. When designing studies, every attempt should be made to minimize distress, risk, and the number of participants, consistent with good study design.
Per the International Conference Harmonisation guideline E11(R1), ensuring a prospect of clinical benefit is required to justify the risks of exposing children to an investigational product.1 Depending on the anticipated benefit-risk balance of an intervention, sponsors often need to have adequate reassurance from adult studies (typically Phase 2) that this balance is favorable before initiating studies in pediatric patients by understanding the drug pharmacokinetics (PK)/ pharmacodynamics (PD) and response compared with placebo. Adequate adult data are typically required before finalizing pediatric study designs and initiating pediatric studies by virtue of the Pediatric Research Equity Act. It is evolving that FDA is including adolescents in Phase 3 trials. The lack of coordinated use of extrapolation for safety and efficacy amongst global regulatory agencies impacts the timelines and development of intelligent clinical trial designs. Perception of lack of incentive and initiative on the industry side, unlicensed use, insufficient return on investment, and enrollment concerns remain active issues.
This has slowed pediatric clinical trial execution to a crawl, with an average delay of 7.5 years and 7.7 years from adult to pediatric labeling approval of biologics for certain diseases like ulcerative colitis (UC) and Crohns disease (CD), respectively.2 The advances in gene therapy development require the collaboration of global regulatory agencies to balance benefit and risk and ensure that decisions that delay drug development do not ultimately prevent the therapies from reaching the needed population of children awaiting cures. The regulatory standards supporting the use of extrapolation of efficacy include the role of pediatric extrapolation as defined in the International Conference Harmonisation E11(R1) guideline.1 This is “an approach to providing evidence in support of effective and safe use of drugs in the pediatric population when it can be assumed that the course of the disease and the expected response to a medicinal product would be sufficiently similar in the pediatric target and reference (adult or other pediatric) population.”2 Extrapolation of pediatric efficacy has a specific legal definition in the United States, relying on fundamental assumptions: “If the course of the disease and the effects of the drug are sufficiently similar in adults and pediatric patients, [FDA] may conclude that pediatric effectiveness can be extrapolated from adequate and well-controlled studies in adults, usually supplemented with other information obtained in pediatric patients, such as pharmacokinetic studies.”2 The European Medicines Agency guidance on extrapolation describes that “sufficiently similar” is not a black and white definition; there may be uncertainties associated with the data supporting extrapolation to the target pediatric population. The extrapolation approach should address these uncertainties, using clinical judgment to establish what level of uncertainty is acceptable. Whilst efficacy extrapolation may be supported, ultimately safety assessments are required for the pediatric population and the extent of this safety data will vary.
Unfortunately, the guidance does not delineate specificity for gene therapies. It is clear that the paradigm in gene therapy is unique, for example, the adult form of the disease may not be relevant for understanding disease pathobiology or manifestations in children. Often there is a later onset that has a different disease course; safety may not be relevant if there is meaningful disease progression, and it could actually be more unsafe to dose adults (see the cRISPR DMD case study). 3,4 Ethical Underpinnings and Regulatory Review The extrapolation issue is particularly relevant in constructing an ethical framework to allow investigation of potential drug candidates in children before adults. In scenarios such as central nervous system degenerative and neurodevelopmental disorders, intervention earlier in the disease progression may influence the natural history of the disease. Development of therapeutics targeted to the needs of children mandates that first-in-human trials involve children. The ethical basis for justification of children first can be complicated by extrapolation in diseases where the disease presents in childhood and the patients live into adulthood. In diseases that are neurodevelopmental affecting both children and adults, complications of the disorder progress with age and by adulthood result in a nonconsenting adult who cannot willingly participate in clinical trials.
For the nonconsenting adult who is not protected under Subpart D- Additional Safeguards for Children in Clinical Investigations creates a scenario that mandates earlier testing in children.3 In children, the disease manifestations are at an earlier point of development and delaying pending completion of clinical trials places the child at further therapeutic orphan status. Therefore, the lack of ability to use extrapolation from adult efficacy and safety data mandates earlier intervention in pediatric subjects. For clinical trials involving children as subjects, IRBs must review and approve only those clinical investigations that agree with the criteria under 21 CFR part 50 subpart D, as detailed in Table 1 below and implemented in the Spinal Muscular Atrophy (SMA) case study.3
Demonstration of safety and documentation of the drugs effectiveness are critical. Therefore, the benefit-risk assessment is integrated into FDA’s regulatory review of investigational and marketing applications. In cases where serious risks are predictable, FDA may conclude a favorable risk profile if the drug clearly demonstrates direct and meaningful benefit on the most important clinical outcomes for a serious or life-threatening disease or it could be determined that the drug represents a specific important advantage over currently available therapies. The Draft Guidance for Industry – Benefit-Risk Assessment for New Drug and Biological Products (September 2021) discusses FDA’s approach to benefit-risk assessment for new drugs and biologics and outlines their benefit-risk framework for new drug review. This framework is a multi-dimensional approach for identifying, assessing, and communicating the important factors in FDA’s benefit-risk assessment. The Agency considers several dimensions, including analysis of condition and the current treatment options, followed by product specificity for assessing benefit, and risk and risk management. There are two important elements to each dimension:
1) The evidence and uncertainties that are relevant to the analysis of condition, benefit-risk assessment, and current treatment options.
2) The conclusions and reasons based on the strength and potential significance of that evidence
The final benefit-risk overview integrates the evidence and uncertainties about a drugs benefits and risks and considers them in the context of the disease severity and current unmet medical needs.4
Regulatory Considerations for Gene Therapy and Rare Diseases
FDA takes into consideration that a higher degree of uncertainty exists for drug development programs studying rare diseases, as limitations in study size can limit precision in safety and efficacy characterizations. There could be greater regulatory flexibility in certain programs with clinical trials that have lower sample sizes or evaluation of sensitivity of effect.5 Especially in these cases, it can add tremendous value if sponsors initiate benefit-risk planning early in development and ensure frequent interactions with the Agency. It is important to note that benefit-risk assessment does not end with the approval of a drug. Benefit-risk assessment is a lifecycle approach, realizing that our understanding of the product’s benefits and risks changes as new information becomes available.4 Development of gene therapy poses unique challenges and opportunities on benefit-risk assessment in children. Due to the nature of most diseases that are amenable to treatment with gene therapy, the potential promise of efficacy by correcting the underlying cause of disease mandates new paradigms for consideration. The success of ZOLGENSMA® (onasemnogene abeparvovec-xioi) is a particularly important example of paradigm shift. ZOLGENSMA® is an adeno-associated virus vector-based gene therapy indicated for the treatment of pediatric patients less than 2 years of age with spinal muscular atrophy with bi-allelic mutations in the survival motor neuron 1 (SMN1) gene (https://www.novartis.com/us- en/sites/novartis_us/files/zolgensma.pdf).
Table 1: 21 CFR 50 Subpart D
The ability for a one-time treatment with ZOLGENSMA® to extend the lives of babies and allow them to continue reaching developmental milestones, is indeed a success for children with SMA, but there are also risks posed by gene therapy. There have been gene therapy treatments that have resulted in deaths in trials affecting children with Duchennes Muscular dystrophy and X-linked myotubular myopathy (DMD and XMTM). Understanding the benefits and risks of gene therapy for vulnerable children is critical to understand and define during the development process for these new innovative therapies. This manuscript helps to define the benefit-risk framework that should be considered for gene therapies.
Case Study: Spinal Muscular Atrophy
Spinal Muscular Atrophy is a group of hereditary disorders that affect the central nervous system, peripheral nervous system, and voluntary muscle movement (skeletal muscle). The most common form of SMA is caused by homozygous loss of function mutations of the Survival Motor Neuron 1 (SMN1) gene on chromosome 5q.6 There are different types of SMA that are caused by mutations in the SMN1 gene that present with a wide range of impairment, from onset before birth with breathing difficulties at birth to mild weakness in adults. The most severe is SMA type 1, also called Werdnig- Hoffman disease or infantile-onset, with symptoms including hypotonia, diminished limb movements, lack of tendon reflexes, fasciculations, swallowing and feed difficulties and impaired breathing. Without any treatment, most children with SMA type 1 do not survive past two years of age due to respiratory failure.7–9 Spinal Muscular Atrophy type 2, also called Dubowitz disease, is an intermediate form of the disease that presents between ages 6 to 18 months with individuals being able to sit without support but unable to stand and walk. The progression of the SMA type 2 is variable and individuals live into adolescence or young adulthood.
There are two milder forms, SMA type 3, also known as Kugelberg-Welander disease, and SMA type 4 which both have normal life expectancy. Individuals with SMA type 3 may present with some muscle weakening during childhood but often can walk and stand without assistance. Over the progression of the disease, individuals may lose those functions and require a wheelchair. Spinal Muscular Atrophy type 4 usually begins in early adulthood with individuals experiencing muscle weakness, tremors and mild breathing problems.10 Spinal Muscular Atrophy type 1 is an attractive candidate for gene therapy because it is a monogenic disease. Efficacy studies in mice that were treated intravenously with a self- complementary AAV9 (scAAV9) containing the SMN1 gene had a significant extension of life to 250 days compared to GFP treated animals that did not survive past 22 days.11 In order to dose pediatric patients in a first-in-human study, 21 CFR 50, subpart D specifically 21 CFR 50.52 needed to be addressed (Table 2).
Table 2: 21 CFR 50 Subpart D
The regulatory development of ZOLGENSMA® utilized a first-in-human pediatric trial and subsequent pivotal trial to accelerate the approval of a one-time treatment of the most severe form of SMA. Currently, additional SMA trials are being performed to assess a different route of administration (STRONG, NCT03381729) and also treatment of the pre-symptomatic SMA type 1 and 2 (SPR1NT, NCT03505099).14
Case Study: Pediatric Neurodevelopmental and Inborn Errors of Metabolism Disorders
15–17To develop the benefit-risk calculus for a first- in-human trial in pediatric participants with neurodevelopmental disorders affecting children and adults, several factors need to be assessed including the ethical framework as it relates to 21 CFR 50, subpart D specifically 21 CFR 50.52, the Agency’s current thinking on prospect of direct benefit, and the precedence of AAV gene therapies being studied in a pediatric population in advance of adults.
The components of a benefit-risk calculus for first- in-human gene therapy treatment for pediatric patients needed to integrate the ethical framework underlying investigation in children under 21 CFR 50.52,18 as well as consideration of
Table 3: 21 CFR 50 Subpart D
Table 4: First-in-Human Gene Therapy in Children with Diseases that Affect Both Populations
Table 5: Ethical Framework Underlying Prioritization of Pediatric Participants for the First-in-Human Trial
a ICH E11(R1) pediatric extrapolation guideline: “an approach to providing evidence in support of effective and safe use of drugs in the pediatric population when it can be assumed that the course of the disease and the expected response to a medicinal product would be sufficiently similar in the pediatric target and reference (adult or other pediatric) population.” Legal definition of extrapolation of pediatric efficacy in the US: “If the course of the disease and the effects of the drug are sufficiently similar in adults and pediatric patients, [FDA] may conclude that pediatric effectiveness can be extrapolated from adequate and well-controlled studies in adults, usually supplemented with other information obtained in pediatric patients, such as pharmacokinetic studies.” (21 CFR §355c)
The FDA generally has defined prospect of direct benefit based on evidence to support the proof of concept, typically derived from multiple data sources (e.g., in vitro mechanistic studies, in vivo studies in translationally relevant animal disease models, clinical studies in adults if appropriate, or previous studies in children), and on the structure of the study intervention (e.g., dose selection and duration of treatment as specified in the clinical protocol). The balance of nonclinical data must support the safety of the proposed administration to a pediatric subject for the proposed paradigm to be justified from analysis of the benefit-risk calculus.
The established unmet medical need supports intervention in pediatric participants to avoid long- term complications and ameliorate disease course. Correction of defective gene function early in the course of disease may maximize the probability of prospect of direct clinical benefit to study participants by correcting a key epigenetic regulator of neurodevelopment before the accumulation of irreversible clinical sequelae occurs. Early correction offers the highest probability of a prospect of benefit, addressing early complications compromised during the initial period of rapid regression and identified as central concerns of caregivers. A trial design should be directly protective of the safety of the human pediatric subject through appropriate safety monitoring by an independent DSMB and the proposed long-term follow-up study. The pediatric trial should be conducted with outcome measures to assess whether the study drug is benefitting the individual child and to support judgments about prospect of direct benefit to guide subsequent studies assessing how a child feels, functions and survives.
Conclusions
The paradigm of pediatric drug development can be multi-dimensional as evidenced by the broad overview presented here. The statutory requirements to study children in clinical trials are influenced by the nature of the disease that is currently under study and needs to be personalized. Shirkey has noted that “If we are to have drugs of better efficacy and safety for children, those responsible for childcare will have to assume this responsibility for developing active programs of clinical pharmacology and drug testing in infants and children. The alternative is to accept the status of “Therapeutic Orphans” for their patients.”20 The mandate of the 21st century is to heed these words; there is no better evidence that the advances in pediatric drug development in gene therapy have been heralding and heeding this mandate.
Conflicts of Interest Statement
All authors are employees of Neurogene Inc. and subject to receipt of stock grants when awarded. There are no conflicts of interest in regard to this manuscript.
Acknowledgement
The authors gratefully appreciate the perspectives and review by Rachel McMinn, PhD for continued thoughts of this manuscript.
References
1. Research C for DE and. E11 Clinical Investigation of Medicinal Products in the Pediatric Population. U.S. Food and Drug Administration. Published April 29, 2020. Accessed February 6, 2023. https://www.fda.gov/regulatory- information/search-fda-guidance- documents/e11-clinical-investigation- medicinal-products-pediatric-population
2. Mulberg AE, Conklin LS, Croft NM, I-ACT for Children Pediatric Extrapolation Working Group. Pediatric Extrapolation of Adult Efficacy to Children Is Critical for Efficient and Successful Drug Development. Gastroenterology. 2022;163(1):77-83. doi:10.1053/j.gastro.2022.03.018
3. Only Patient in N-of-1 CRISPR Trial for Duchenne Muscular Dystrophy Dies. BioSpace. Accessed July 14, 2023. https://www.biospace.com/article/patient-in- n-of-1-crispr-trial-for-duchenne-muscular- dystrophy-dies-/
4. TaylorMay 19 NP, 2023 09:35am. DMD gene therapy death exposes risks of treating older patients. Fierce Biotech. Published May 19, 2023. Accessed July 14, 2023. https://www.fiercebiotech.com/biotech/dmd- gene-therapy-death-exposes-risks-treating- older-patients
5. 21 CFR Part 50 Protection of Human Subjects. Accessed February 6, 2023. https://www.ecfr.gov/current/title- 21/chapter-I/subchapter-A/part-50
6. Research C for DE and. Benefit-Risk Assessment for New Drug and Biological Products. U.S. Food and Drug Administration. Published September 29, 2021. Accessed February 6, 2023.
https://www.fda.gov/regulatory- information/search-fda-guidance- documents/benefit-risk-assessment-new-drug- and-biological-products
7. CFR - Code of Federal Regulations Title 21. Accessed February 6, 2023. https://www.accessdata.fda.gov/scripts/cdrh
/cfdocs/cfcfr/CFRSearch.cfm?fr=312.80
8. Lefebvre S, BÜrglen L, Reboullet S, et al. Identification and characterization of a spinal muscular atrophy-determining gene. Cell. 1995;80(1):155-165. doi:10.1016/0092- 8674(95)90460-3
9. Finkel RS, McDermott MP, Kaufmann P, et al. Observational study of spinal muscular atrophy type I and implications for clinical trials. Neurology. 2014;83(9):810-817. doi:10.1212/WNL.0000000000000741
10. Kolb SJ, Coffey CS, Yankey JW, et al. Baseline results of the NeuroNEXT spinal muscular atrophy infant biomarker study. Ann Clin Transl Neurol. 2016;3(2):132-145. doi:10.1002/acn3.283
11. Kolb SJ, Coffey CS, Yankey JW, et al. Natural history of infantile-onset spinal muscular atrophy. Annals of Neurology. 2017;82(6):883-891. doi:10.1002/ana.25101
12. Spinal Muscular Atrophy Fact Sheet | National Institute of Neurological Disorders and Stroke. Accessed January 27, 2023. https://www.ninds.nih.gov/spinal-muscular- atrophy-fact-sheet
13. Foust KD, Wang X, McGovern VL, et al. Rescue of the spinal muscular atrophy phenotype in a mouse model by early postnatal delivery of SMN. Nat Biotechnol. 2010;28(3):271-274. doi:10.1038/nbt.1610
14. Mendell JR, Al-Zaidy S, Shell R, et al. Single- Dose Gene-Replacement Therapy for Spinal Muscular Atrophy. New England Journal of Medicine. 2017;377(18):1713-1722. doi:10.1056/NEJMoa1706198
15. Day JW, Finkel RS, Chiriboga CA, et al. Onasemnogene abeparvovec gene therapy for symptomatic infantile-onset spinal muscular atrophy in patients with two copies of SMN2 (STR1VE): an open-label, single-arm, multicentre, phase 3 trial. Lancet Neurol. 2021;20(4):284-293. doi:10.1016/S1474- 4422(21)00001-6
16. Jablonka S, Hennlein L, Sendtner M. Therapy development for spinal muscular atrophy: perspectives for muscular dystrophies and neurodegenerative disorders. Neurol Res Pract. 2022;4(1):2. doi:10.1186/s42466-021- 00162-9
17. Downs J, Stahlhut M, Wong K, et al. Validating the Rett Syndrome Gross Motor Scale. PLoS One. 2016;11(1):e0147555. doi:10.1371/journal.pone.0147555
18. Neul JL, Kaufmann WE, Glaze DG, et al. Rett Syndrome: Revised Diagnostic Criteria and Nomenclature. Ann Neurol. 2010;68(6):944- 950. doi:10.1002/ana.22124
19. Fu Y, Zhan X, Wang Y, et al. NLRC3 expression in dendritic cells attenuates CD4+ T cell response and autoimmunity. EMBO J. 2019;38(16):e101397. doi:10.15252/embj.2018101397
20. Mulberg AE, Murphy D, Dunne J, Mathis LL. Pediatric Drug Development. John Wiley & Sons; 2013.
21. Bhatnagar M, Sheehan S, Sharma I, et al. Prospect of Direct Benefit in Pediatric Trials: Practical Challenges and Potential Solutions. Pediatrics. 2021;147(5):e2020049602. doi:10.1542/peds.2020-049602
22. Shirkey H. Therapeutic orphans. Pediatrics. 1999;104(3 Pt 2):583-584.
Author Area
Have an article to submit?
Submission Guidelines
Submit a manuscript
Become a member